Looking Beyond Neurons
by Lindsay Borthwick
Research highlights from Kavli Neuroscience Institutes
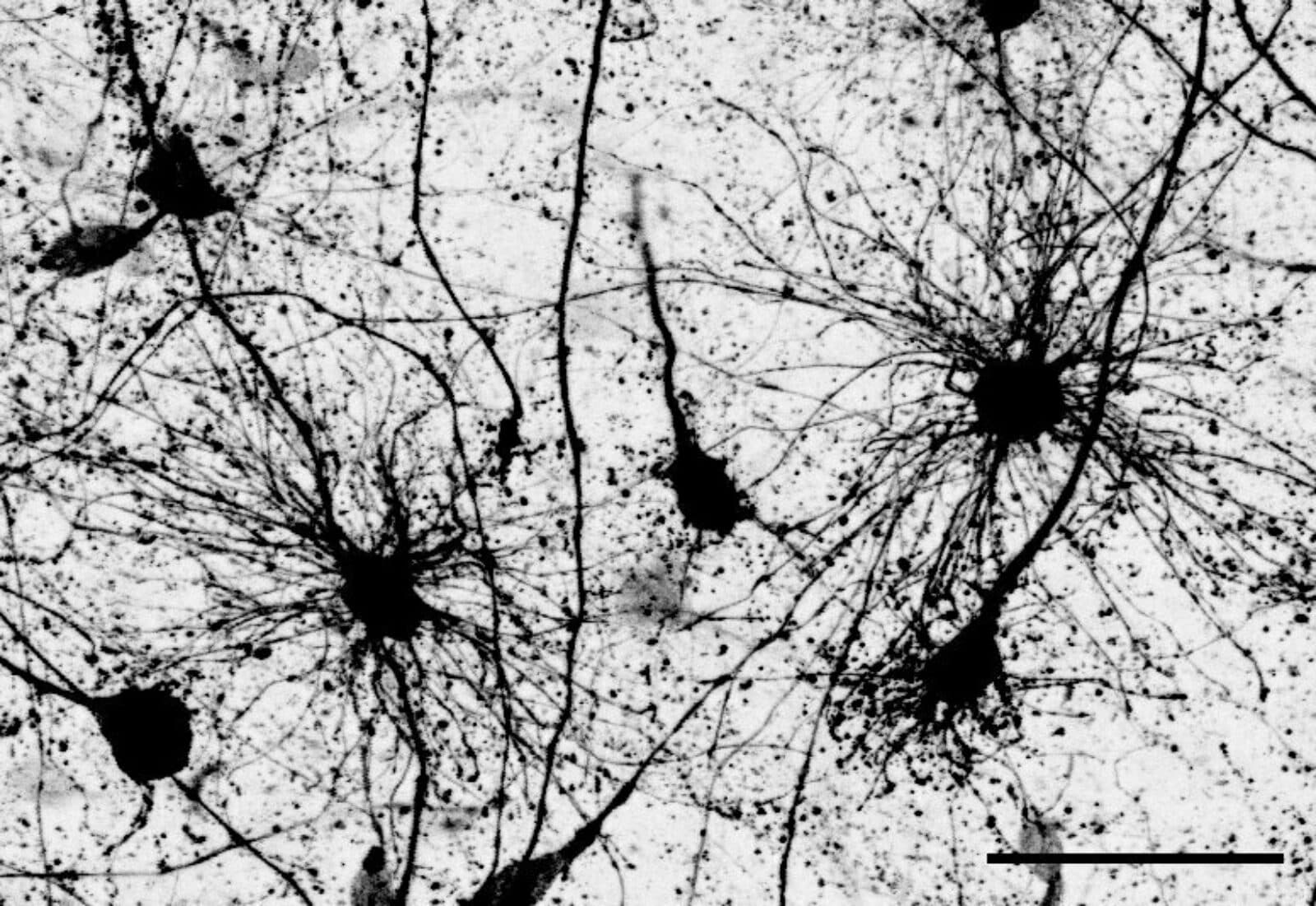
The Author
When we think about the nervous system, we usually think of neurons — the cells that conduct electrical impulses from the tips of our toes to the brain and through the web of connections within it, generating movement and coding our thoughts. But neurons aren’t the only cell type in the brain. For example, there are star-shaped astroglia that vastly outnumber neurons, and microglia — immune cells that reside in the brain. All three of these cell types factor into new discoveries from researchers at Kavli Institutes, highlighted in this round up. In studying these cell types, Kavli researchers are expanding our view of the brain and the roots of neurological and psychiatric disease. Then there are plants. By definition, they don’t have a nervous system. Yet, a new study raises the possibility of using them as a model system to study psychiatric diseases. Read on to learn more about the latest Kavli research.
A star-spangled cortex
In the developing human brain, radial glia are the immature cells that give rise to astrocytes — star-shaped cells that are actually far more numerous than neurons. Scientists have shown that radial glia diverge into two populations midway through gestation. What happens next is the focus of a new study by neuroscientist Denise Allen, a doctoral student in the lab of Tomasz Nowakowski at the University of California, San Francisco (UCSF). Allen and Nowakowski investigated the steps that occur as neural stem cells mature and multiply, eventually forming the cerebral cortex, where billions of astrocytes and neurons are organized into different layers and regions. For a long time, astrocytes were thought to be support cells in the brain. Now, neuroscientists are increasingly interested in how they may contribute to brain health and neurological diseases including schizophrenia, bipolar disorder and autism. When the UCSF researchers tracked the development of the two populations of radial glial cells, they found that the cells give rise to two distinct subpopulations of astrocytes: one subgroup that resides in the brain’s white matter; another in the grey matter. In addition to location, the astrocytes also differ in shape and molecular identity. The study is important for understanding fundamental properties of brain development. Dr. Nowakowski is a member of the Kavli Institute for Fundamental Research at UCSF.
The roots of mental health
Can we study the brain in an organism that doesn’t have one? What about psychiatric illnesses like schizophrenia, that alter that behavior? It’s an audacious idea — one that Tamas Horvath, a neuroscientist affiliated with Yale’s Kavli Institute for Neuroscience, has been exploring for close to a decade. Horvath studies how the brain controls fundamental processes like metabolism, usually in mammals like mice. Now, in newly published research, he is testing his ideas in a small, flowering plant called Arabidopsis thaliana. He focused on a mitochondrial gene, Friendly Mitochondria (FMT), that is found in the plant, along with a homologous gene, Clustered mitochondria homolog (CLUH), found in mice. His interest in mitochondria stems from the fact that they are the energy-producing structures within cells. Furthermore, dysfunctional mitochondria have been implicated in neurodegenerative and psychiatric diseases like Alzheimer’s and schizophrenia. The study’s goal was to compare the genes’ roles in plants and animals. Next, Horvath and his team plan to look at other metabolic genes and biological processes that are conserved across species and compare their functions. “The long-term goal is to develop a sort of dictionary that catalogues these similarities between plants and animals and to use it to ask research questions more robustly,” Horvath told Yale News. “It’s possible this plant can serve as a complementary model organism for behavioral research in the future.”
Seeing learning up close
As we learn, new neuron connections form in the brain. But how do those new connections lead to changes in the brain and our behavior? That is the question at the heart of a new paper in Nature Neuroscience from the lab of Takaki Komiyama, a member of the Kavli Institute for Brain and Mind at the University of California, San Diego, and led by Dr. Nathan Hedrick. The team used a combination of imaging techniques to investigate the formation of learning-induced dendritic spines — small protrusions on neurons that receive input from other neurons — their survival, and resulting brain function. In mice, they observed new spines form near clusters of existing spines, which were previously active during a motor learning task (pressing a lever in response to hearing a specific noise). The newly formed spines that ultimately survived were those that coupled their activity with existing spines. By being active together, clusters of spines give a boost to new, learning-related synapses. These observations suggest that spines involved in learning are selectively maintained. In a Tweetorial about the new paper, Hedrick explains that the new spines seldom share axons with other spines in a cluster, suggesting that learning involves binding new information streams together into shared functional synaptic clusters.
Stemming synaptic loss in Alzheimer’s Disease
Developing an effective Alzheimer’s drug has proven to be extremely difficult. Most drug candidates have targeted the buildup of beta-amyloid plaques in the brain—a telltale sign of the disease. Dr. Stephen Strittmatter, director of the Kavli Institute for Neuroscience at Yale University, is taking a different approach. His lab recently tested an experimental drug that prevents activation of the immune system, the idea being that preventing chronic inflammation associated with the buildup of beta-amyloid in the brain may prevent the death of neurons in the end stages of the disease — and the cognitive declines that come with it. When the compound was tested in two mouse models of Alzheimer’s, brain synapses and cognitive function were restored in the mice. The drug actually reversed certain gene expression changes in neurons, and prevented activation of proteins that trigger inflammation. If it could be administered to patients early enough, he suggests, it may help prevent the dramatic loss of synapses that leads to the disease’s most devastating symptoms. Dr. Strittmatter is founder of Allyx Therapeutics, a biotech company that is testing the drug in early stage human trials. Dr. Jason Cai, assistant professor of radiology and biomedical imaging at Yale, is co-corresponding author on the study, which was published in Science Translational Medicine.
Making sense of time
How do humans gauge the time at which an event occurred, such as the time we left for work? Do we rely on an internal clock that somehow guides those estimations, or is it a psychological effect? In a new study, Drs. Jacob Bellmund and Christian Doeller combined brain imaging with learning tasks that required participants to memorize a sequence of events and reconstruct the times at which the events occurred. As the sequences unfolded, the researchers used a hidden clock that was occasionally revealed to cue participants to the passing time. Sometimes the time was correct, whereas other times the clock was sped up or slowed down relative to real time. The researchers found that psychologically constructed time shapes our memories more so than the time that actually elapses. The findings suggest that as the brain constructs episodic models, such as the sequences of events, the brain combines various pieces of information to gauge time. Rather than relying on an internal clock that somehow stamps the time on these memories, we construct the time at which events occur based on other information. For example, we might estimate the time we left for work based on whether or not we heard the morning news or caught the bus at our regular time. The research adds to our understanding of memory and may help us understand memory impairment in diseases like Alzheimer’s. Doeller is a visiting group leader the Kavli Institute for Systems Neuroscience at the Norwegian University of Science and Technology and Managing Director of the Max Planck Institute for Human Cognitive and Brain Sciences.