How Global Change Tests the Limits of Neural Systems
by Lindsay Borthwick
Scientists are beginning to unravel the impact of anthropogenic change on the neural systems of species as diverse as fish, honeybees, and ground squirrels.
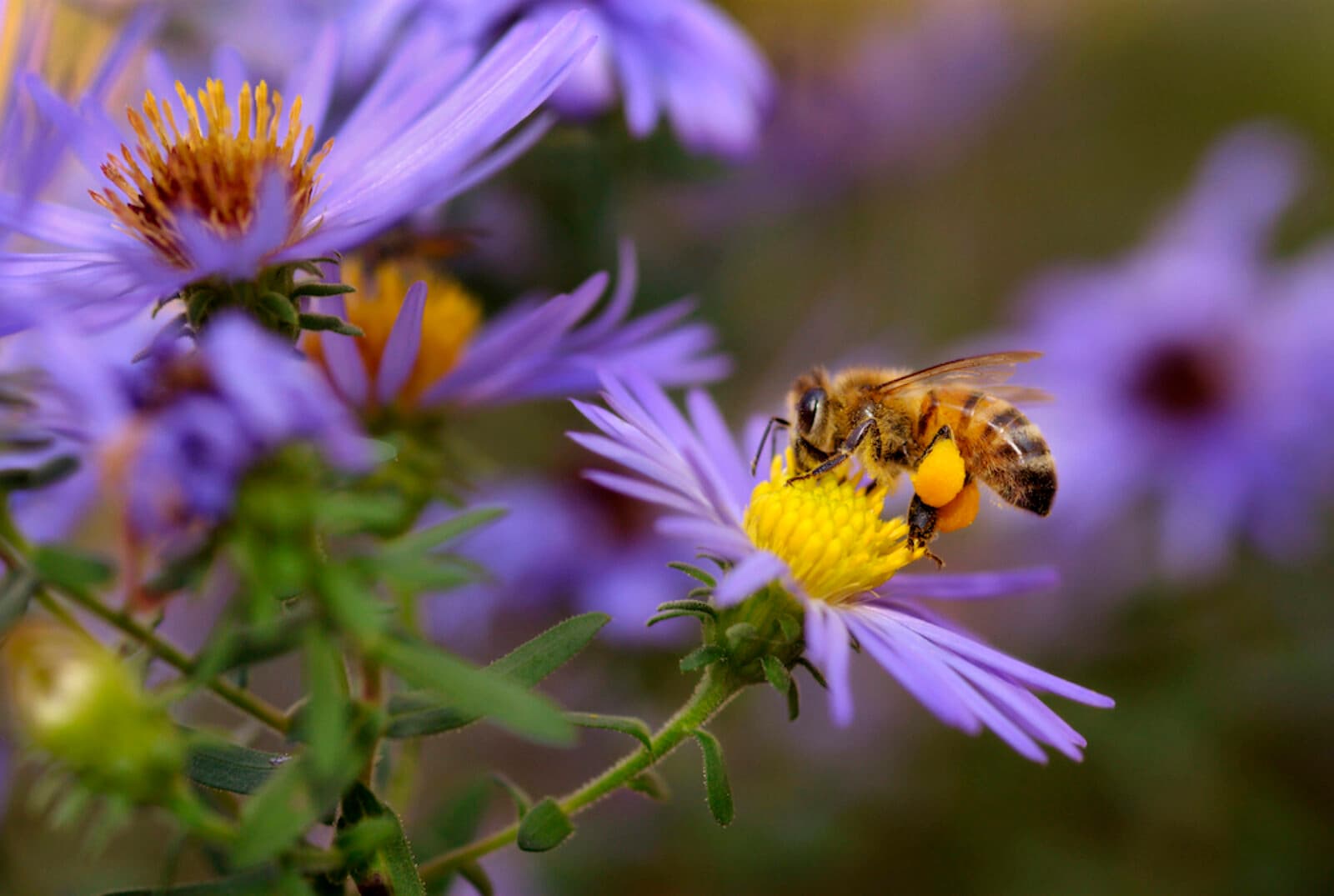
The Author
The past winter was the warmest on record in the United States, a whopping 3°C hotter than the long-term average. Maple sap began flowing early. Insects were active sooner. And, bears began emerging early from their winter torpor.
It’s a natural experiment that is being emulated in the lab by neuroscientist Elena Gracheva, who studies hibernation - not in bears, but in ground squirrels. At the Kavli Institute for Neuroscience at Yale University, she brings nature into the lab, a state-of-the-art hibernation facility, where Gracheva can precisely control the squirrels’ living conditions, including light, temperature, humidity, and the availability of food and water. She is using hibernation to uncover how the nervous system adapts to an organism’s state. It is an extreme example of environmental and behavioral change - a state at the very limit of mammalian physiology.
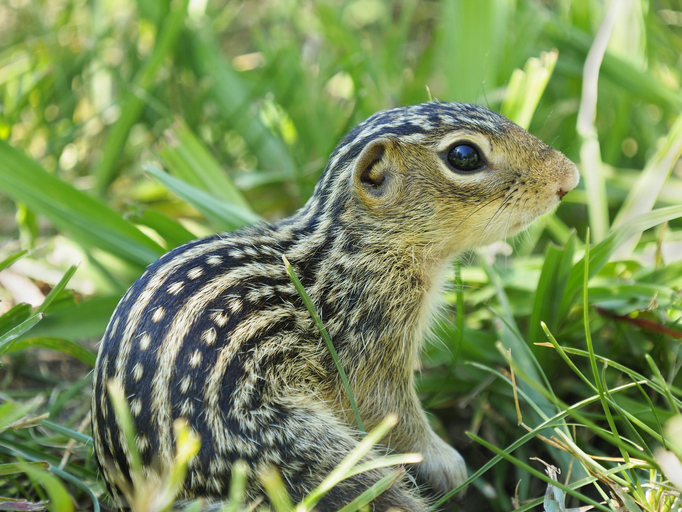
Gracheva’s research is an example of the emerging field of neurobiology and changing ecosystems. Scientists are asking how the neural systems of animals are impacted by anthropogenic changes such as climate change - and the fluctuations in temperature and seasonality that come with it – and other phenomena like ocean acidification and light pollution. Their goal is to understand how nervous systems endure the challenge of rapid, human-induced environmental change, and how they adapt.
For Gracheva, that means, what are the neural mechanisms that regulate a ground squirrel’s body temperature, which plunges from 37°C to less than 5°C during hibernation? How is neural activity restored as an animal’s body warms up in spring? As winters warm, disrupting hibernators’ finely tuned evolutionary adaptations, how will they respond?
More broadly, researchers forging this new field are investigating whether the nervous system can adapt to the challenges of the anthropocene, and, if so, what will that plasticity look like at the molecular, cellular, circuit, and organismal level? Can we predict what might happen in five, 50 or 100 years in response to various stressors? And, can we learn from some animals how to help others adapt to environmental extremes, including ourselves?
The timing couldn’t be more urgent. Records for the hottest, wettest, driest, or most polluted places on Earth are routinely being broken with potentially devastating consequences for diverse ecosystems and the species that depend on them.
“We need to be creative and take advantage of new technologies. This is the time for us to look at the problem of climate change and do comparative neurophysiology across different species,” says Gracheva.
As Gracheva suggests, in some ways, the timing also couldn’t be better. The field is building on a century of discovery about how neural systems function, and increasingly powerful research tools make it possible to probe neural systems at multiple levels, including molecules, cells, and circuits, and integrate that new knowledge.
The result could be a much richer understanding of how neural systems function and how their built-in plasticity allows organisms to withstand environmental stressors and adapt.
For this to happen, science also needs to undergo a metaphorical transformation from winter to summer, in which connections are forged between the bench and field, between researchers studying model and non-model species, and between disciplines.
Catalyzing a new kind of science
Jeff Riffell is a biologist at the University of Washington (UW) in Seattle, whose research on olfaction in insect pollinators has taken him from the humid environments of Florida to arid environments across the Western U.S. In his latest study, published earlier this year in the journal Science, Riffell and UW atmospheric chemist, Joel Thornton, paint a global picture of how nighttime pollution disrupts pollinator activity. They showed that nitrate radicals, released into the air by cars and power plants, destroy the scent cues that guide nighttime pollinators to wildflowers. This disrupts the plant-pollinator interactions that are so critical to the health of ecosystems. They also used data on global weather patterns and atmospheric chemistry to identify the places in the world where pollination would be most impaired by pollution.
Riffell is among an increasing group of scientists linking neuroscience, ecology and global change. He says individuals working in ecology or conservation have recognized the need for the past 10 or 20 years. Now, the neuroscience community is awakening to it. “I think there's a growing understanding that there has to be a link between the two because, ultimately, the nervous system is controlling behavior, and we're seeing these really strong anthropogenic effects on the behavior. So, there has to be this link,” he says.
Like Riffell, Mar Huertas, a physiologist at Texas State University in San Marcos, Texas, also studies olfaction. However, Huertas works with a variety of fish species - from zebrafish, a model organism critical to neuroscience research, to catfish, the basis for the largest aquaculture industry in the U.S. She is studying how exposure to low but persistent levels of nitrogenous compounds in surface water affect fish's sense of smell at the molecular and cellular level, their brain function, and their behavior.
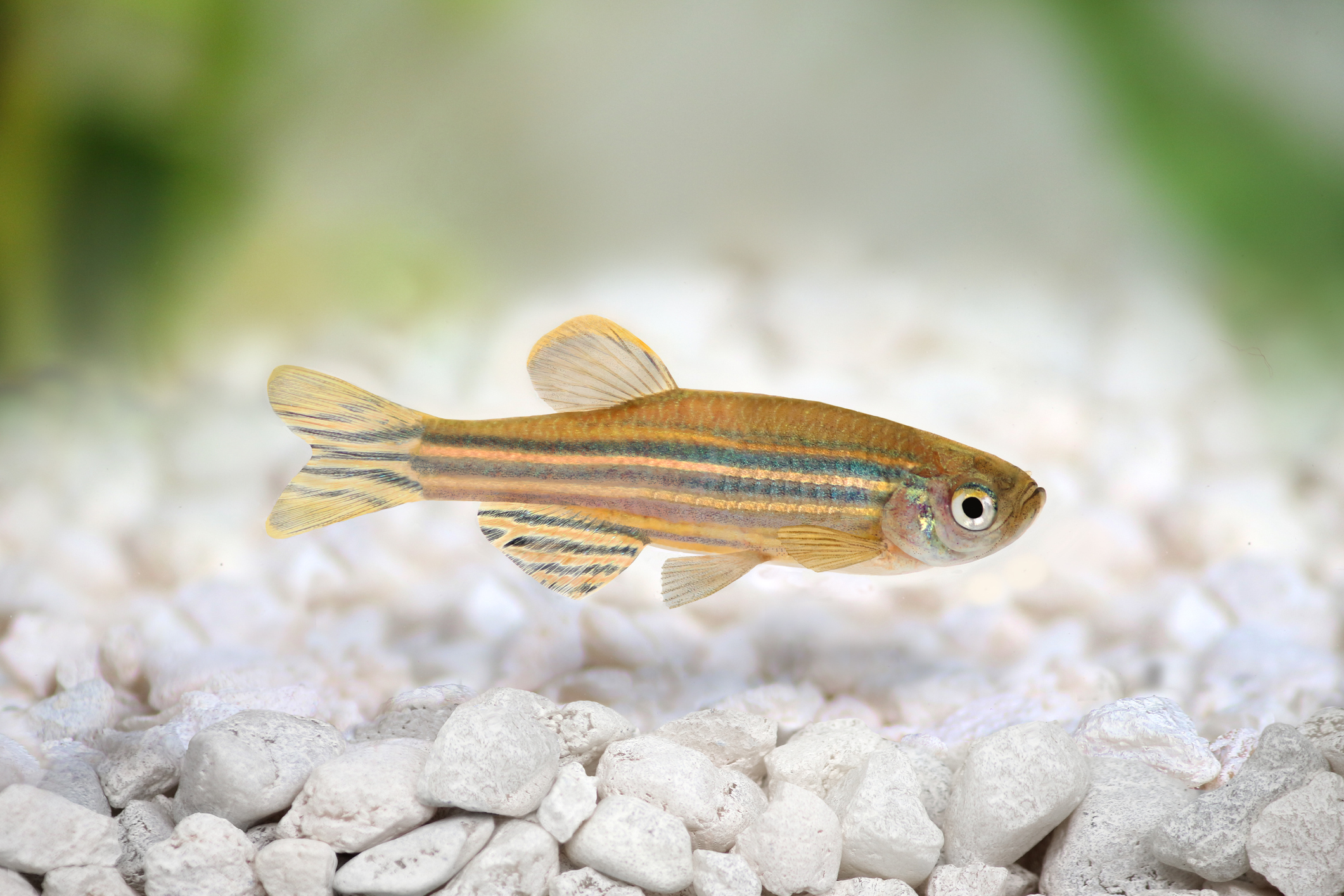
“We know what [these chemicals] do at high concentrations, but not at very low concentrations, like the ones we can find now in rivers. In fish, you have all these neurons in the nose exposed to the water. They are a portal to the brain, so it’s very likely that constant exposure to these chemicals affects their physiology and, ultimately, their health,” says Huertas.
Levels of nitrites and nitrates are rising in the water supply globally and climate change is also shifting the nitrification process toward nitrite, which is more toxic. This has serious implications for animal and human health. In fact, in the U.S., she says, nitrite/nitrate levels often exceed acceptable concentrations for drinking. Huertas has discovered that the chemicals impair the fish’s ability to smell and their ability to find food. They also stimulate the animal’s immune system and disrupt their microbiome, which may affect brain function.
“We’re not sure whether the loss is due to sensory or cognitive changes,” says Huertas. “We’re now trying to understand the neural mechanisms, and also to look at human health as well when they drink these compounds every day.”
Given that her research has critical implications for human health, aquaculture, and the environment, Huertas says there is a need for better connection not only between researchers in the lab and in the field, but also between researchers and policymakers.
Creating real-world impact
In her lab at Oxford University, Rachel Parkinson is also thinking about how to ensure the latest science on sensory pollutants and their impact on animals is factored into government decisions.
Parkinson completed her Ph.D. in neuroethology, the study of the neural basis of animal behavior, at the University of Saskatchewan in Saskatoon, the heart of Canada’s breadbasket. At that time, her focus was on locusts, an agricultural threat. Currently, she is studying pollinators like honeybees - an essential and irreplaceable part of modern agriculture. She is unpacking how sublethal levels of pesticides affect the insect’s visual and taste perception and their ability to navigate and interact with food sources.
“We're able to both look at the effects that pesticides are having on these insects, so that we can get a better understanding what they might be experiencing in the field, but also to use these compounds as pharmacological tools to dig into the sensory biology, the circuits, and understand more about how they work,” says Parkinson.
She says scientists need to figure out what pesticide concentrations affect insect behavior and how, so regulators can make better decisions about the chemical being used in the environment.
“We know pesticides are toxic. That is no surprise. We also know that climate change and nutritional stress are bad and likely confounding the effects of pesticides and other pollutants. But we need to find ways to more easily, more reproducibly, and more scalably look at these effects so that we can do something about them,” says Parkinson.
She is currently developing an automated system that takes advantage of computer vision and machine learning to study the effects of pesticides and other environmental stressors on insect behavior. She hopes it will speed up discovery across species and environmental stressors and make it easier to translate research findings into policy.
Martin Tresguerres, who studies how marine organisms sense the environment and how their senses are affected by ocean acidification, agrees. He sees an opportunity to create research centers or interdisciplinary research programs dedicated to the neurobiology of changing ecosystems, where non-model organisms like fish, honeybees, ground squirrels and other ecologically important species take center stage. Research would focus on establishing how the neural systems work in diverse species, how they adapt to the environment under normal conditions, and how they are affected by anthropogenic environmental change.
It’s a bold vision that hinges on the development of new tools and resources for studying neural systems in non-model organisms. “The cells are different. You cannot use the same templates or systems for these other organisms,” says Tresguerres, a marine biologist at the Scripps Institution of Oceanography in San Diego, California. “It comes down to this: How can you look at the effects of climate change on something when you don't know how it works to begin with?”
He also says interdisciplinary collaboration and a sustained, coordinated effort to look at neural systems and their adaptations from genes to molecules to cells to organisms to populations will be critical to advancing the field.
“That is something that is essential. Now, you can identify genes in a population that are different under certain environmental conditions. But to really know what those genes are doing, you need cell biologists. And then, to know whether these changes are relevant for the animal you need the integrative animal physiologists and the behavioral people.”
These observations underscore that as human-driven changes reshape the planet, science also has to adapt. It must evolve to deliver the knowledge needed to accurately predict and rapidly respond to a whole new world.