How French Fries Talk to the Brain and Other Discoveries
by Lindsay Borthwick
Research highlights from Kavli Neuroscience Institutes
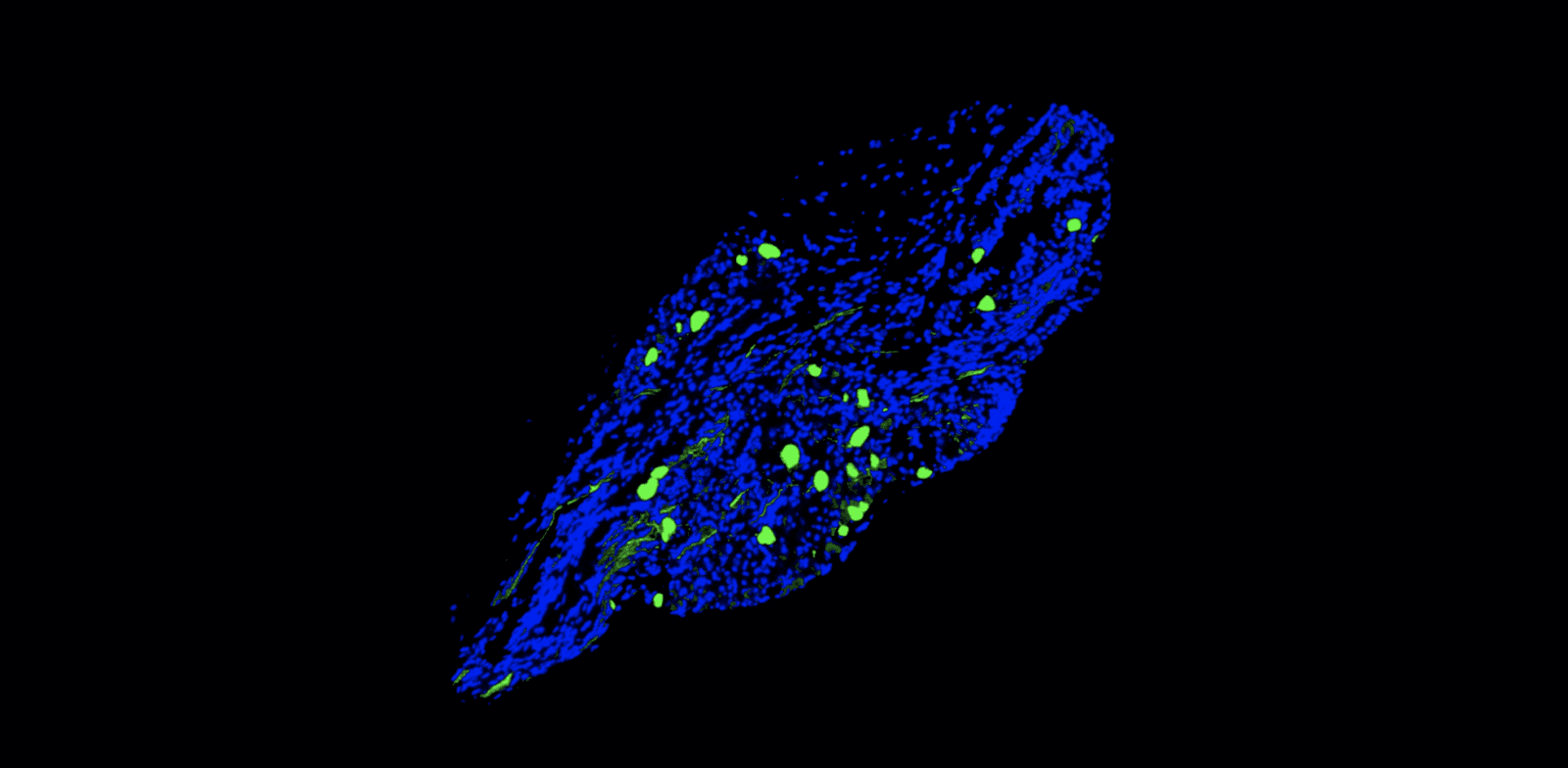
The Author
This roundup of research highlights from different Kavli Institutes span neuroscientific discoveries from head to toe. Often overlooked, the peripheral nervous system contains nerve cells that carry instructions to muscle cells via the neuromuscular junction, or NMJ. New research from the Kavli Institute at UCSF extends research observations from the NMJ into the mammalian brain, suggesting there are similar mechanisms at play. Then there’s the exploration of sensory receptors and circuits in the gut, which is sometimes called the body’s “second brain,” and the power it has over our appetite and food cravings. Finally, research on ‘jumping genes’ expressed by nerve cells in the “little brain” — the cerebellum — suggests they are involved in a rare, genetic form of ataxia and could be important to our broader understanding of other neurological disorders.
Your cravings, explained
“The gut is the source of our great desire for fat and sugar,” according to Charles Zuker, a neuroscientist at Columbia University where he is member of the Kavli Institute for Brain Science and a Howard Hughes Medical Investigator. Zucker knows a thing or two about the biology behind our appetite for all things sweet and fatty. A few years ago, he led a team of researchers that showed how sugar in the gut drives our cravings for sweets. Together with Dr. Mengton Li and other colleagues, he shows that fat fuels similar cravings. A series of experiments in mice showed that the preference for fatty foods like French fries and bacon doesn’t come from the mouth. Instead, it occurs well after ingestion, once fat hits the gut. The team also identified the fat-sensing circuits between the gut and the brain, including receptors that are triggered by fat and by nutrients essential to survival.
Brain mapping gets a boost
Neuroscientists are celebrating a $500-million injection of funds into U.S. National Institutes of Health's Brain Research for Advancing Innovative Neurotechnologies (BRAIN) Initiativeto support an ambitious brain mapping effort. The goal is to map the brain cells and circuits across multiple species, especially humans. The new effort builds on more than a decade of research funded by the BRAIN Initiative, which was launched as a private-public partnership by former president Barack Obama.
The funding will launch The BRAIN Initiative Cell Atlas Network (BICAN) and the Armamentarium for Precision Brain Cell Access. BICAN will build cell atlases for non-human primates and the human brain, building on past research dedicated to mapping the mouse brain. The Armamentarium funding is dedicated to creating new tools to help researchers study brain activity at the cellular and circuit levels.
“[The brain] is a highly complex organ whose connections and organizational principles we do not come close to understanding, and there was a realization that we needed better tools,” John Ngai, Director of the NIH BRAIN Initiative told STAT News in an article about the announcement.
In addition to being the non-Federal co-chair of the BRAIN Initiative Alliance, The Kavli Foundation is a longstanding supporter of the BRAIN Initiative and its vision to drive brain research forward through fundamental research and the development of new tools to make that research possible. Kavli-affiliated researchers at the University of California, San Francisco, Rockefeller University, Johns Hopkins University and Yale University are some of the many neuroscience researchers who are funded through this initiative.
The stable brain
How the nervous system stays stable throughout life, maintaining our capacity to learn and remember, is the focus of Graeme Davis’s research at the University of California, San Francisco (UCSF). Just as the body adapts to maintain our balance on new terrain, nerve cells adapt to maintain their ability to signal other cells in the nervous system as we’re confronted with new experiences. In a recent paper, Davis and his team identify some of the important biochemical processes involved in presynaptic homeostatic plasticity, or PHP — a major way nerve cells adjust and maintain their function. The research provides a framework for understanding how PHP is triggered and how it progresses. It also identifies the key molecules involved in PHP in both fruit flies and mice. A second paper from the lab confirms that a process resembling PHP also occurs in the mammalian brain. The lab’s ultimate goal is to understand how this ancient and fundamental process works in humans, and how it contributes to diseases of the nervous system such as epilepsy, autism, post-traumatic stress disorder and psychosis. Davis is the co-director of the Kavli In stitute for Fundamental Neuroscience at UCSF.
Mind, brain, behavior...
At the heart of neuroscience is understanding the connection between the mind, the brain and behavior. Two studies out of San Diego demonstrate the power of science to illuminate that connection. The first was partly funded by the Kavli Institute for Brain and Mind (KIBM) at the University of California, San Diego. Davide Dulcis, a professor of psychology, shows that bright light therapy, which is used to treat mood disorders, can trigger nerve cells in the brains of mice to switch the expression of neurotransmitters. That switch leads to changes in brain activity and the animal’s daily behaviors. The findings helps explain the therapeutic effects of bright light therapy for seasonal affective disorder and other, non-seasonal, mood disorders.
A second study, led by Kenta Asahina at the Salk Institute, pinpoints a gene and a group of cells in fruit flies involved in de-escalation, the ability to decide when it’s time to stop fighting. What causes aggressive behaviors is pretty well understood, whereas less research has been focused on what triggers an animal to stop fighting. “We’ve found an important mechanism in the brain that normally prevents us from expressing high levels of aggression,” Asahina says in a news article. “Although our findings are in fruit flies, the same mechanism may be at play in humans, at least at the molecular level, which could help better explain a host of psychiatric diseases.” Asahina is a member of the Kavli Institute for Brain and Mind at the Salk.
Jumping genes in the spotlight
Jumping genes — so named because these stretches of DNA can move — are abundant in the human genome. In fact, they make up whopping half of it. They perform a variety of functions, especially during brain development, but are generally silenced in adulthood. However, sometimes they can cause the onset of rare, genetic diseases such as ataxia-telangiectasia, a deadly movement disorder. Yale research scientist Takehiro Takahashi, working with co-senior investigators Akiko Iwasaki and Tomas Horvath, studied LINE-1, a common jumping gene, in the cerebellum, and whether it caused the genetic disorder. Working in mice, he found that activating LINE-1 in cerebellar neurons caused DNA damage, cell death, and deterioration of the animals’ movement over just a few weeks. Remarkably, he was able to prevent the progression of the disease using a reverse transcriptase inhibitor – the class of drugs used to treat HIV. The research points to a new cause of movement disorders and raises the possibility that jumping genes could be involved in other neurological diseases. “This study illustrates the danger of LINE-1 dysregulation in the brain, and highlights that neurological diseases can have their origins in transposons and viral infections, Takahashi told Yale News. Horvath is a member of the Kavli Institute for Neuroscience at Yale.