Celebrating Diversity and Discovery in Neuroscience
by Lindsay Borthwick
Research highlights from Kavli Neuroscience Institutes
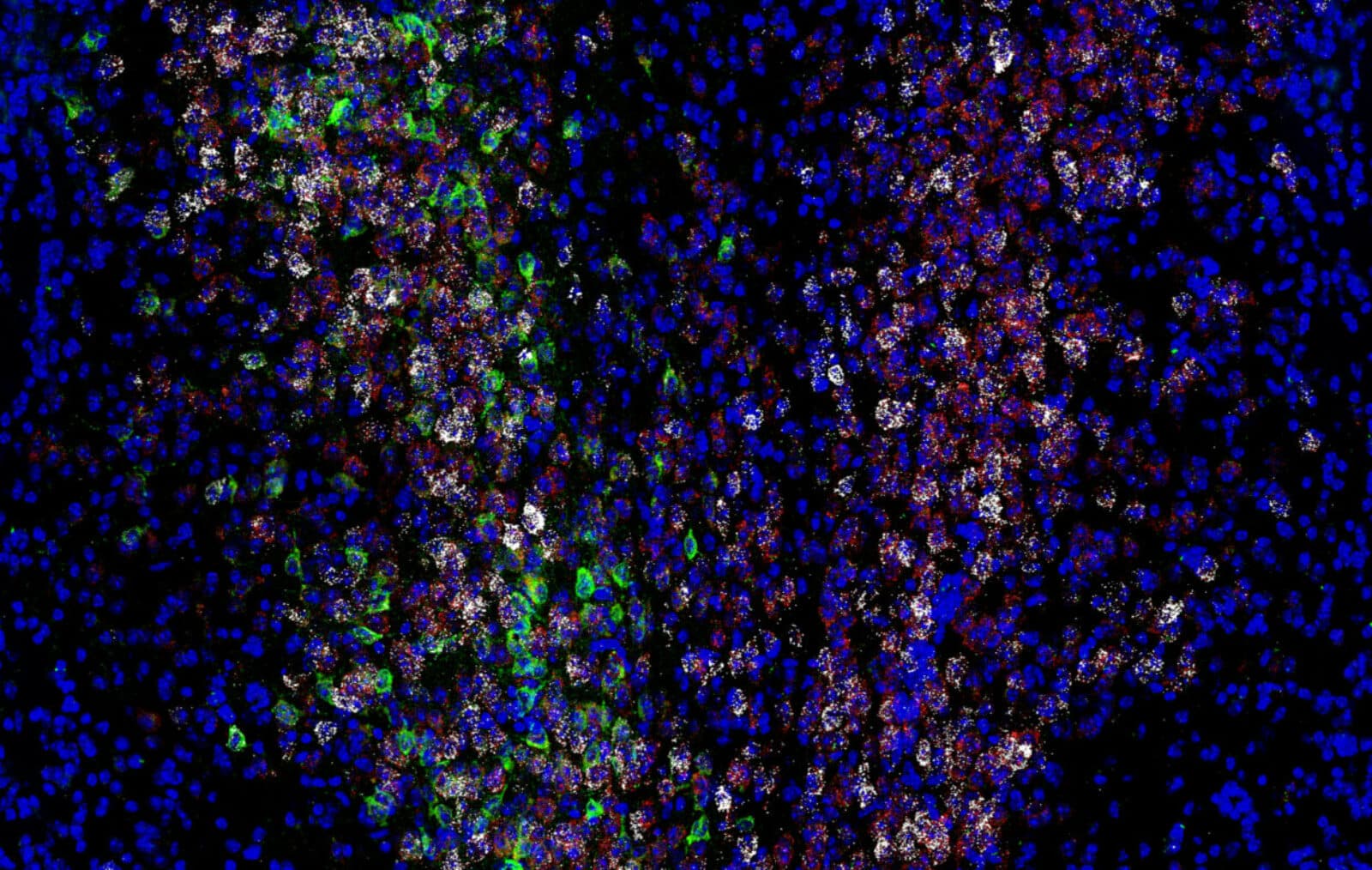
The Author
The latest research highlights from the Kavli Neuroscience Institutes range from insights into memory that reveal new pathways dedicated to recalling past events to molecular changes that may be at the root of Parkinson’s disease. The research itself is important, but so too is the environment in which it happens. The Kavli Institutes are designed to promote collaboration between scientists with diverse expertise and experiences and to train the next generation of neuroscientists. Some of these early career researchers joined July’s Black In Neuro Week 2022 to celebrate Black scholars and professionals in neuro-related fields. Read on to meet them and learn about their scientific interests.
Celebrating #BlackInNeuro
Neuroscience in July went out with a big finish with Black in Neuro Week 2022! Researchers worldwide joined the #BlackInNeuroRollCall and shared their career journeys in neuroscience-related fields, including some scholars and professionals affiliated with Kavli Neuroscience Institutes. Here are a few to get to know:
- Lauren Neal - a 3rd-year Ph.D. student at Rockefeller University. Lola is studying "how the brain and ovaries of MOSQUITOES communicate to regulate their biting behavior" in the lab of Leslie Vosshall, former director of the Kavli Neural Systems Institute. Neal was just awarded a Gilliam Fellowship for Advanced Studies from the Howard Hughes Medical Institute, supporting students from historically excluded and underrepresented populations striving to become future scientific leaders.
- Austin Coley, Ph.D. - a postdoctoral fellow with Kay Tye, a principal investigator at the Salk Institute and a member of the Kavli Institute for Brain and Mind. Coley studies the effects of neural circuits on behavior and how changes in those circuits relate to neuropsychiatric conditions. He is also one of 40 contributors to a new book, Success Tips from Successful African Americans.
- Rafael Perez, Ph.D. - a Kavli postdoctoral fellow at the Kavli Institute for Neuroscience (KIN) at Yale University. Perez works in the laboratory of KIN Deputy Director Marina Picciotto, where he studies associative learning in opioid addiction. "I aspire to investigate how biology interacts with the challenges that plague minority communities and drive disparities in substance use disorder outcomes. My goal is to combine basic science with translational research to resolve these critical questions," said Perez, who received the Kavli Postdoctoral Award for Academic Diversity last December.
- Martin Rosenfeld - a postgraduate research associate in Pietro De Camilli's lab, which is associated with the Kavli Institute at Yale. Rosenfeld, who is also a member of the Department of Genetics' Diversity Advisory Committee, said he wants to send a message to future Black leaders in science that, "We are not only capable of forging our own destiny, but we can make an impact on all those who follow us."
- Caitlin Corona-Long - a 5th-year doctoral student in Michaela Gallagher's lab at Johns Hopkins University who studies "fluid & MRI biomarkers of the earliest stages of Alzheimer's Disease." Corona-Long said she is a first-generation Ph.D. student born to a Sierra Leonian father and an American mother. Outside the lab, she puts her scientific skills to work studying her family tree. Gallagher is a member of the Kavli Neuroscience Discovery Institute at Hopkins.
Curiosity Quenched
When we eat something delicious, especially foods containing fat and sugar, brain cells release dopamine, reinforcing those food choices. A new study found that dopamine also helps guide an animal toward hydrating foods, which are just as crucial for survival. Zachary Knight, a professor of physiology at the University of California, San Francisco (UCSF) and a member of UCSF's Kavli Institute for Fundamental Neuroscience, led the research. Knight's team wanted to understand how animals learn what to eat in the wild, particularly fluids and rehydrating foods. When an animal gulps water, the mouth immediately signals the brain. However, it takes time for water to enter the bloodstream and then for the brain to register the resulting changes in fluid balance. So, the researchers let lab mice freely drink water and recorded neural activity in individual neurons in an area of the midbrain called the ventral tegmental area (VTA) over an extended period. They observed two waves of neural activity in dopamine neurons in the VTA — one active wave as mice drank and a second wave about 10 minutes later when fluids entered the blood. When the researchers silenced the dopamine neurons active during the second wave, the mice could not learn the connection between the fluid source and hydration. The findings suggest that subsets of dopamine neurons track internal signals to drive learning about rehydrating foods.
Total Recall
Priya Rajasethupathy and other neuroscientists are overhauling the idea that individual memories are stored in discrete spots in the brain, waiting to be retrieved. Instead, they have shown that memory processing is a dynamic process involving large numbers of neurons located in different parts of the brain. Dr. Rajasethupathy's latest study, recently published in Nature, underscores the distributed nature of memory processing. It also illuminates how animals retrieve memories — and what disparate parts of the brain are involved in memory recall. In a series of experiments, Rajasethupathy's team showed that cross-talk between the hippocampus, which is essential for forming and storing memories, and the prefrontal cortex (PFC), a brain region long-associated with higher cognitive functions like decision-making and attention, is vital for memory retrieval. The researchers used a virtual reality setup to train mice to associate different rooms with sensory cues that were either good or bad. As the mice explored their environment, the researchers observed the neural activity in various parts of the animals' brains. They found that as mice formed memories, sensory information associated with those memories was stored in the PFC. Later, that information could be used to trigger the global memory in the hippocampus. The findings show that the mammalian brain has a pathway dedicated to memory recall that is separate from memory formation. Rajasethupathy is associate director of the Kavli Neural Systems Institute at Rockefeller.

Bitter Memories
Kay Tye's
research team at The Salk Institute for Biological Studies is investigating the brain circuitry underlying emotion and motivation, with the goal of developing targeting treatments for brain disorders such as anxiety, depression, and post-traumatic stress disorder. In a recently published paper in Nature, they showed how the brain assigns a positive or negative emotional value, or valence, to memories. The findings are valuable because they may lead to a better understanding of why some people tend to retain negative emotions rather than positive ones, particularly when they are depressed or struggling with other mental health conditions. The researchers tested the role of a neuropeptide called neurotensin in associating good and bad feelings with a memory. When they eliminated neurotensin signaling in a specific set of neurons, mice could not assign a positive valence to an experience, such as associating a sweet taste with a particular tone. The absence of neurotensin did not affect negative valence, such as associated a bitter taste with a tone. This finding suggests the brain defaults toward fear or avoidance, a state that promotes survival from an evolutionary viewpoint. "We can actually manipulate this switch to turn on positive or negative learning," says co-first author and postdoctoral fellow Hao Li in a news article. "Ultimately, we'd like to try to identify novel therapeutic targets for this pathway." Tye is a member of the Kavli Institute for Brain and Mind.

Unpacking Parkinson's Disease
Parkinson's disease has been difficult to tackle due to challenges in understanding all the cellular pathways that are involved in disease progression. Pietro De Camilli, former director of the Kavli Institute for Neuroscience at Yale University, and his team have identified numerous genetic mutations associated with the disease and are studying how they impact brain cells. Two recent studies focused on the Vps13c gene, whose mutations lead to a hereditary form of Parkinson's that develops early in adulthood. In the past, the researchers discovered that the VPS13C protein forms a bridge between two subcellular compartments: the endoplasmic reticulum, which regulates the production of fatty molecules that help form the cell membrane, and lysosomes, which collect and dispose of waste within cells. Now, the researchers have zoomed in on the structure of the protein and confirmed that it helps transport lipids between the two compartments in healthy human cells. A separate study investigated how Parkinson's-associated mutations in the Vps13c gene affect nerve cells. They found that a lack of the VPS13C protein in human cells affects the cell's lipid composition and the properties of lysosomes. Furthermore, these changes activate the innate immune system, the body's first line of defense. The finding raises the possibility that Vps13c mutations may trigger inflammation in brain tissue, which may be a process that impacts Parkinson's Disease.