Tightening the Bounds on the Biggest Thing There Is, Dark Energy
by Adam Hadhazy
Part 5 of our 'Looking Ahead to Rubin' series takes in dark energy's grandness and its even grander mysteriousness, both of which will be attended by the upcoming Legacy Survey of Space and Time.
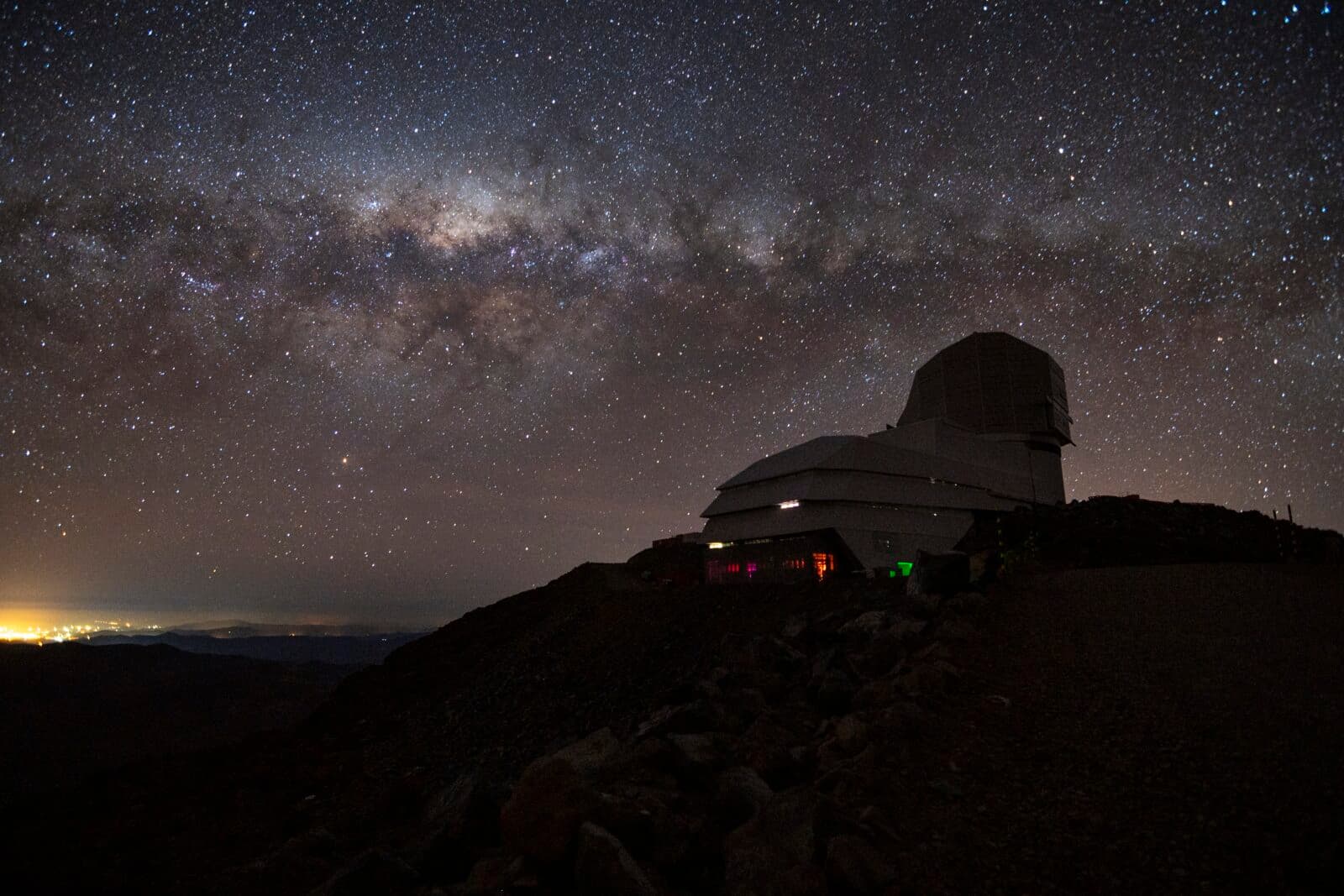
The Author
Our understanding of the dynamics of the universe is about to be revolutionized, thanks to the Vera C. Rubin Observatory. Come 2024, this observatory is slated to begin its Legacy Survey of Space and Time (LSST), a campaign that will observe the full sky every three nights over the course of a decade. The survey will capture an unprecedented amount of astrophysical data, opening our eyes to the cosmos as never before.
Groups of researchers worldwide have banded together in forming science collaborations to provide scientific expertise for the Rubin Observatory. Many Kavli Institute-affiliated researchers belong to these science collaborations. This article is part of a series profiling these researchers and the advances they expect the groundbreaking observatory will deliver.
Suppose a scientist from another universe with its own distinct cosmic composition, dynamics, and laws of physics were able to visit our universe—what sort of impressions might this being get?
Assuming this traveling scientist beheld our universe in its current epoch, approximately 14 billion years after the Big Bang, the scientist would assuredly overlook us infinitesimal Earthlings, along with anything small and insignificantly planetary, or even stellar in nature. Instead, the scientist might logically first focus on the dominant entity on hand—the entity we refer to as dark energy.
Various lines of evidence have all converged on dark energy's oversized presence. From observations of distant stellar explosions, to the chronology of galactic cluster structure formation, to even the big bang's relic light, all signs point to dark energy. Altogether, this invisible entity is strongly evidenced to compose about two-thirds of the mass-energy of the universe. Stated another way, and implausible as it sounds, this intangible dark energy is the lion's share of everything that exists. Perhaps stranger still, this dark energy appears to be accelerating the expansion of the universe, and has been doing so going back eons.
Like our fictional traveler, we nonfictional humans understandably have a lot of questions about this thing called dark energy. Unfortunately, though, at this time we have no definitive answers. The hope is the Rubin Observatory LSST will at last pry open the black box, if you will, of dark energy.
Dedicated to this task is the Dark Energy Science Collaboration (DESC) for the Rubin Observatory LSST. Katrin Heitmann, the spokesperson for DESC, summarizes why she and her colleagues are hopeful about the survey's prospects thusly: "The survey has been often described as 'wide, fast, deep' and that is exactly what LSST will bring to the table: an incredible data set of tens of billions of stars and galaxies over an area much larger than previous surveys were able to capture and at more depth," Heitmann says. "Mapping out the galaxies and with it the structures of the universe and the growth of those structures is unprecedented at the scales LSST targets. It will provide us with an incredible amount of information about the make-up and the history of the universe."
Charting that history and gauging the universe's composition more accurately will help in nailing down the properties of dark energy as never before. As Heitmann explains, some of the proposed explanations for dark energy so far include a "cosmological constant," a sort of negative gravity or energy inherent to the near-vacuum of space; a universe in which Albert Einstein's general theory of relativity—our best explanation of gravity—does not hold on the largest, cosmological scales; or that there is some dynamical origin to dark energy that remains unglimpsed.
"The data promises to guide us in addressing these questions," says Heitmann, who is a physicist and computational scientist at the Argonne National Laboratory as well as a member of the Kavli Institute for Cosmological Physics (KICP) at the University of Chicago.
Also inherent to the LSST's pursuit will be hoovering up data on dark energy's "dark universe" counterpart, dark matter. This substance has its own strong lines of evidence. Among the most compelling is that some sort of gravity-exerting, yet unobservable material must be present in great quantities in order for galaxies to hold themselves together.
Dark matter is thought to consist of undiscovered particles similar in many ways to the ordinary matter that comprises us, the planets, stars, and everything else we think about when we consider what's "real" and exists. Amazingly, though, dark matter is estimated to outnumber this regular ol' matter about five times over. As with dark energy, powerfully mapping the universe will help us constrain dark matter's properties and thus get us closer to identifying it.
Along the way, LSST can also help us extrapolate back to the universe's origins in the Big Bang while potentially resolving some of the profoundest, still-unresolved mysteries about ordinary matter. One such mystery is the mass of neutrinos, which is so slight that it remains unmeasurable. Still other fascinating, novel insights should also emerge as the survey plumbs the cosmic depths.
Says Heitmann: "[With LSST], we can ask other questions as well, such as what is the nature of dark matter, what happened during the very first moments of the universe—yes, they influence what we see today—[and] how light is the lightest particle we know about, the neutrino? And then of course there might be many surprises that the universe will reveal when we look deeper and deeper and over very large areas of the sky."
The focus of Heitmann's research is computational cosmology, which leverages the processing power of computers to simulate the universe we behold. The universes formulated through computer programs in this way are based on physical laws and behaviors which can be tweaked to hone in on their real, natural values and manifestations. The road goes both ways temporally, so to speak, with simulations explaining what it is that observations have already captured, as well as precisely predicting what it is that new, richly detailed observations should reveal.
"Together with my collaborators, we are using some of the world’s largest supercomputers to provide predictions about the evolution of the universe and signatures for a range of observations," says Heitmann. "With the advent of measurements from the Rubin Observatory, these predictions have to be very accurate to enable the best possible analysis of the data."
Ever-greater precision in both theory and observations has long been a guiding principle for Heitmann, whose background is actually in theoretical particle physics. The discovery of dark energy in the late 1990s, while Heitmann was working on her Ph.D. thesis, intrigued her and inspired a switchover to cosmology. "In both particle physics and cosmology, the major aim is to understand how the universe works at the most fundamental level," Heitmann says. "So it was very natural to move into this area."
Concomitantly then and through today, continuing advances in computing power have been bringing cosmology and other sciences to tremendous new levels. That progress has led to the Rubin Observatory LSST which, in addition to its gargantuan ability to collect data observationally, is being met on the backend by the gargantuan ability to process, store, and analyze that data.
All in all, this dovetailing of curiosity and cutting-edge capability—"a combination of trying to answer fundamental questions and incredible resources both on the observational and computational front"—first drew Heitmann into the cosmological realm and has kept her there since, still seeking.