Seeing Past our Limits and More
by Alan S. Brown
Research highlights from Kavli Nanoscience Institutes
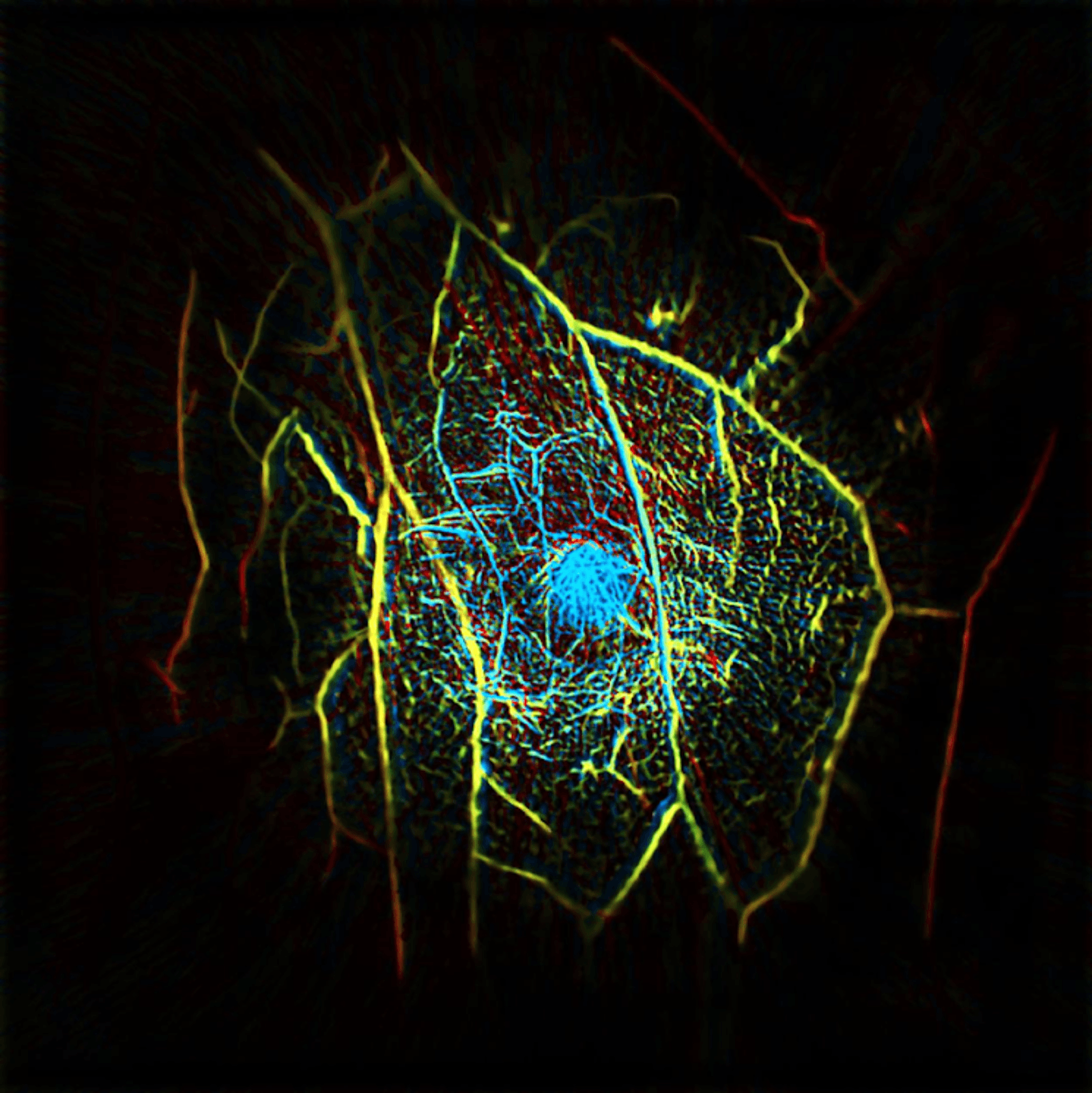
The Author
Recently there has been some fascinating news from Kavli Institutes in Nanoscience. First, scientists found a way to shrink the resolution of 3D-printed features by an order of magnitude, while others learned how computer science explains the way fruit flies keep their balance as they fly. We also see some interesting follow-up research on electron crystals, discover how growing brains on a plate helps address ataxia and other neural diseases, and learn about new technologies enabling us to visualize the nanoscale behavior.
Really small 3D-printed metal parts
3-D metal printing works by laser-melting metal powders to form thin layers that eventually form a finished piece. The smaller the part, however, the harder it gets to create precise features. This is because metals, especially conductive ones like copper and aluminum, transmit laser heat to nearby powders and cause them to melt and solidify. This makes it hard to print parts with resolutions smaller than 100 microns (about the width of a human hair). Now, a team led by Julia Greer, director of the Kavli Nanoscience Institute at Caltech, has developed a way to drive that down to 50 microns or smaller. Instead of printing with metal powders, the researchers create parts from layers of hydrogels, large, flexible polymers that absorb water. Researchers print them one layer at a time and flash them with ultraviolet light to turn them solid. To make metal parts, they dissolve metal salts in the water. The hydrogels then absorb them. When placed in a furnace, high heat burns away the organic material and fuses the metal salts into metal parts. The technique works with a broad range of pure metals and alloys. Greer’s team hopes to use the process to make precise microscale machinery and components for biomedical and scientific devices. One of the developers, Kai Narita, has formed a start-up, 3D Architech, to commercialize the process.

Tugging fruit flies in flight reveals their secrets
A team led by Itai Cohen, a member of the Kavli Institute at Cornell University, is using computer logic to understand how fruit flies remain stable in flight. The researchers used three approaches to probe fruit fly flight. The first, optogenetics, lets them breed fruit flies with neurons that can be can turned on and off by exposure to intense light. This enables control of wing muscles to be ‘on and off’ which allows specific behaviors to be to isolated and linked to individual neuron types. The second involves placing a tiny ferromagnet on the fruit fly. Turning on a magnetic field will tug the fruit fly from its path, forcing it to compensate. The third technology is an 8,000-frame-per-second camera that lets researchers see what happens as they manipulate muscles optogenetically while tugging on the insect. The fly’s control mechanism, it turns out, is similar to the proportion-integral-derivative (PID) controllers that control industrial processes. Flies, like an automated drill or chemical reactor, want to operate at the most energy-efficient realm. When they get pulled by the magnet, they sense how far they are from that point, how long the deviation lasts, and the rate of change. Like the PID, the fly uses this data to right its body and readjust its flight.
Seeing past our limits
A fundamental challenge in nanoscience is visualizing tiny structures. Caltech recently reviewed some of the visualization technologies its researchers are working on. Two were developed by members of the school’s Kavli Nanoscience Institute. Kavli member Mikhail Shapiro, for example, has genetically engineered genes that create gas-filled protein bubbles when activated. Using ultrasound, Shapiro can image when those genes turn on and off deep inside the body. He is using these “acoustic reporters” to track immune cells in the gut microbiome, (where many live) to see how they move through the body to attack pathogens or tumors. Meanwhile, Kavli member Lihong Wang is developing a camera capable of imaging up to 70 trillion frames per second. That is fast enough to freeze light in its path, and he is doing just that to chaotic systems, such as the reflections created by a laser fired into an asymmetrical chamber. With a few modifications, the camera also provided the first images of signals traveling through nerve cells. By combining laser and sound, he also developed a scanner that can pinpoint tumors within 15 seconds without the discomfort or radiation of mammograms.
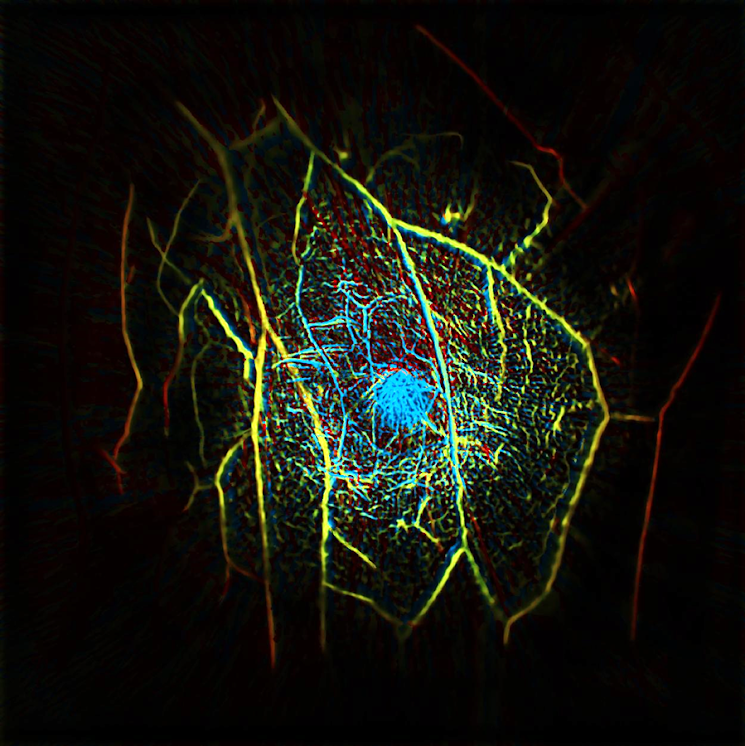
Brains on a plate
When it comes to understanding the genes and biological mechanisms that cause diseases, the brain is biology’s equivalent of a black hole. There is no simple way to collect or observe living human brain tissue to see what’s going on. Our increasing ability to grow brain organoids from human stem cells offers researchers new ways to study the brain. Organoids are small clumps of tissue that can be coaxed to grow some of the same type of neurons found in human brains. At the Kavli Institute for NanoScience Discovery at Oxford University, Esther Becker’s lab has developed a robust protocol to generate tiny organoids that have structural similarity to the cerebellum, the center of the body’s motor coordination. Her team is using these “brains on a plate” to investigate the mutations that cause ataxia, the lack of bodily coordination. In a recent video, a research fellow in her lab, Dr. Jussi-Pekka Tolonen, describes some of the group’s work. First skin cells are converted into stem cells, then induced to differentiate into cells that resemble those found in cerebellum, a process that takes nearly three months. Tolonen’s goal is to modify the stem cells to mimic changes found in cell types vulnerable to ataxia and other cerebellar diseases, then test promising drugs using the organoids as a first step to assessing potential value for therapeutics.