Modified Gravity
by Adam Hadhazy
A theory that could solve confounding cosmic quandaries and unite gravity with the rest of nature's fundamental rules
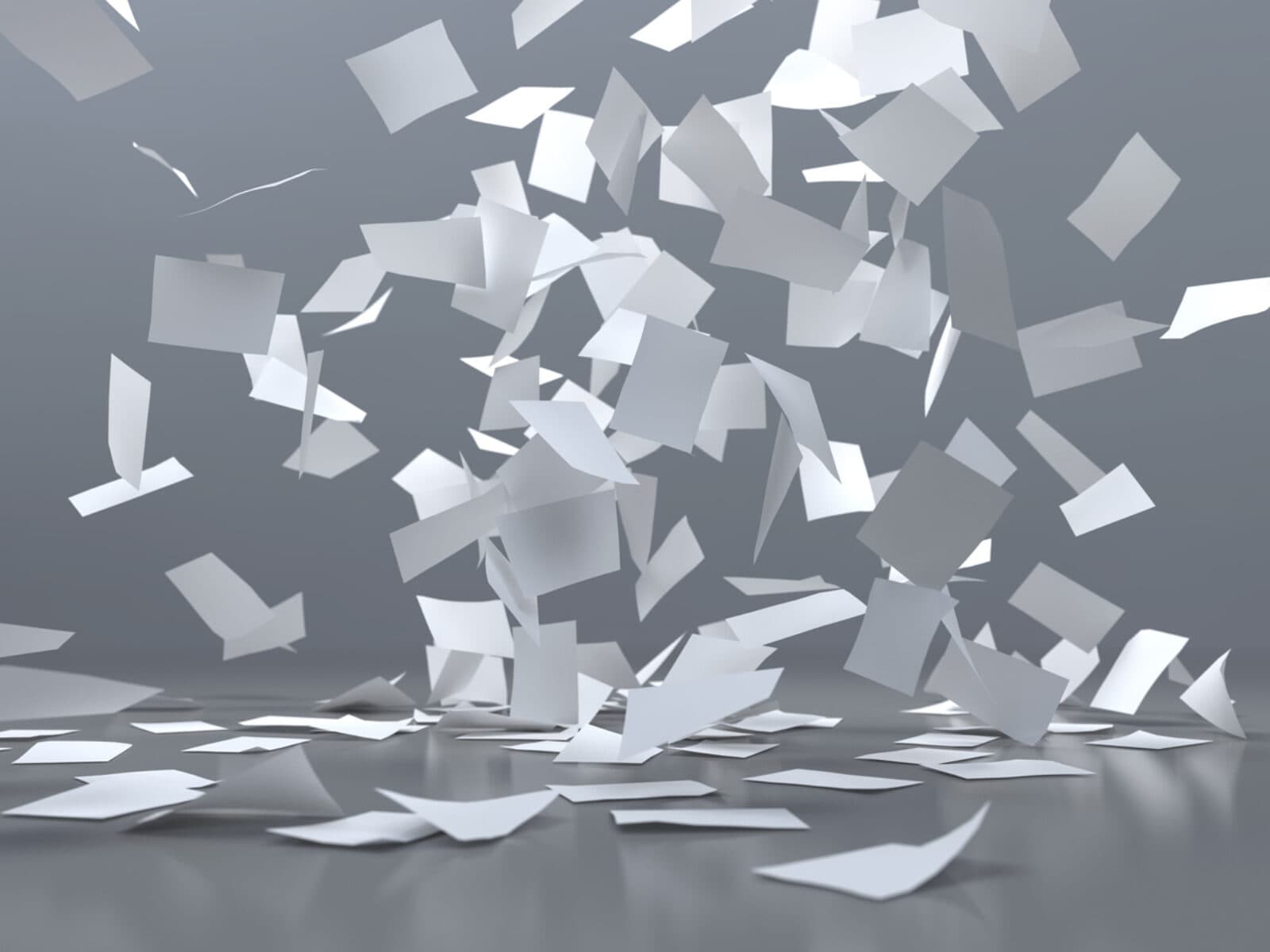
The Author
The Researcher
Of nature's four fundamental forces, gravity is the most innately experienced. From their earliest self-directed moments, tiny children will marvel at how the dropped block — or, to parents' exasperation — the spilled drink falls to the floor. Gravity, we quickly come to learn, is a fact of life, inviolable and unstoppable.
For theoretical physicists, too, gravity is a stubborn thing. Our present explanation for its behavior, the general theory of relativity, has passed every single test we've ever thrown at it. An astonishing array of phenomena that general relativity predicts—including the existence of black holes, gravitational waves, time dilation, gravitational redshift, gravitational lensing, you name it — have all been borne out in the century-plus since Albert Einstein originally postulated it.
Yet general relativity is not the be-all-end-all when it comes to gravitation. For starters, general relativity's gravity does not mathematically mesh with nature's other three forces (namely the strong, weak, and electromagnetic interactions). Zooming out to the universe as whole, general relativity fails to explain severely conflicting measurements of how fast the cosmos is expanding. Nor does it accommodate dark energy, a mysterious, gravity-defying entity comprising around 70% of the universe. The problems don’t stop there. To account for the way galaxies cluster and whirl without flinging their stars into space, theorists have had to slot in another mysterious entity, a gravity-exuding dark matter.
Suffice to say, general relativity is a day late and a dollar short. Faced with these deep dilemmas, some physicists are accordingly pursuing theories of "modified gravity," where hallowed rules can be bent or even broken.
"There are many versions of modified gravity because it can be anything that goes beyond Einstein's theory of general relativity," says Sergei Ketov, who studies modified gravity as the head of the Theoretical High-Energy Physics Lab at Tokyo Metropolitan University and a Visiting Scientist at the Kavli Institute for the Physics and Mathematics of the Universe.
Essentially, modified gravity assumes that on the largest and/or smallest scales, gravity acts in novel ways compared to the well-tested scales in the middle (including those ceaselessly probed by toddlers). "There are now many reasons to expect [general relativity's] modification both for very large distances and for very small distances," says Ketov.
The reasoning behind this thinking is that to date, as Ketov points out, we've only really tested general relativity to ultra-high precision within our own solar system. To name but a couple instances, we've gauged how the four-dimensional spacetime described by the theory influences the orbits of the planets, as well as the spins of gyroscopes aboard Earth-orbiting satellites.
A host of upcoming experiments — some with involvement from Kavli astrophysics institutes — are aiming to expand the precision-testing of gravity outward into the broader cosmos.
"There are now quite many significant observational tests of modified gravity in the planning," says Ketov. "All of them are related to precision measurements of cosmological parameters of inflation, dark energy, dark matter and gravitational waves."
By "inflation," Ketov means the theory which holds that the universe underwent a dramatic expansion during its earliest moment of the Big Bang. This so-called inflation would have left signatures within the relic light from the Big Bang, known as the cosmic background radiation, an which permeates all of space today. The BICEP3 project is currently seeking those signatures, courtesy of a telescope in Antarctica.
Meanwhile, the EUCLID space telescope, slated for launch next year, will precisely measure galaxies' shapes and distances from Earth to stringently test for dark matter's and dark energy's push-and-pull. Still other efforts include the detection of gravitational waves, the ripples in the fabric of spacetime described by general relativity that were first detected in 2015 by the Laser Interferometer Gravitational-wave Observatory (LIGO), a project led in part by members of the Kavli Institute for Astrophysics and Space Research at the Massachusetts Institute of Technology. Two other gravitational wave detectors, Virgo and KAGRA, have since joined the party. These experiments are lifting the hopes of modified gravity researchers that g-wave anomalies will eventually emerge, lending support to models beyond Einstein's theoretical tour de force. A space-based gravitational detector also in development, called LISA, will raise the ante even further.
Whatever new insights may come, Ketov points out that the tried-and-true general theory of relativity could very much remain a part of a broader framework for this thing we call gravity. "Modified gravity does not rule out Einstein's theory," says Ketov, "but it shows its boundaries."
Sorting out gravity's complete nature would clue us in not only to the universe's origins and its past, but also its eons yet to come; we might be able to definitively answer whether the universe will continue to spread out for eternity or come back together ultimately in a Big Crunch.
More relevantly for humankind in the here and now, a full reckoning of gravity would advance our understanding of fundamental physics. That, in turn, could potentially open the door to new technologies and capabilities critical for our species' survival and flourishing someday — maybe — out in the wider galaxy.
"What those modifications precisely [are to general relativity] is the key scientific question in our efforts to understand the universe," says Ketov. "The right answer is going to be crucial for the future of cosmology, astrophysics, and physics in general, and even for our civilization. Space is our future!"