Listening to the Sound of Gene Expression
by Alan S. Brown
With acoustics, Mikhail Shapiro has learned to see how genes create proteins in organs, tissues, and even cells deep within the body
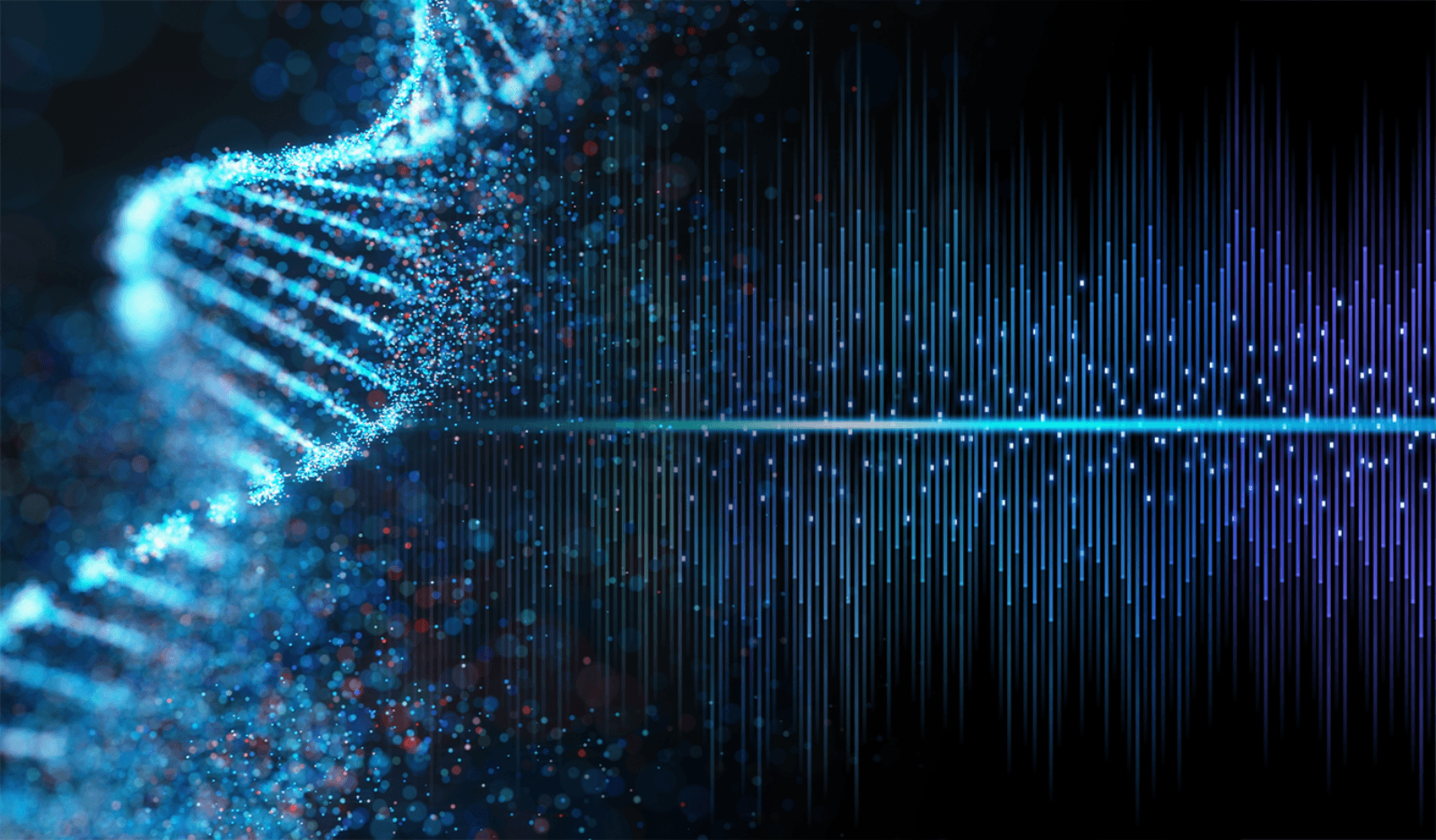
The Author
The Researcher
When it comes to understanding what sparks genes to create proteins in living and cells, our knowledge runs only skin deep. This is because existing technologies to visualize protein formation rely on light, which cannot penetrate more than a millimeter or two beneath the surface. What goes on below is largely unknown.
Mikhail Shapiro, a member of the Kavli Nanoscience Institute at Caltech, where he is a professor of chemical engineering, has developed a way to delve deeper. It involves ultrasound, a technology best known for producing low-resolution images of hardly recognizable growing fetuses. This scarcely taps ultrasound’s true potential as a scientific instrument.
Shapiro’s lab puts ultrasound on steroids, going well beyond anatomy to capture gene expression (the production of proteins) in tissues and even individual cells deep within the body. The research is so potentially transformative, the Howard Hughes Medical Institute (HHMI) awarded Shapiro $9 million in funding over the next seven years to continue his work.
Shapiro’s acoustic sensors turn a well-known visual imaging method on its head. This involves reporter genes, specialized sections of DNA inserted into a gene. When a cell expresses that gene to create a protein, the reporter gene produces a fluorescent protein that shines brightly when illuminated with ultraviolet light. This makes them easy to see and tells researchers when, where, and in what sequence the target proteins are created.
Yet optical reporter genes have a critical limitation: cells in living organisms scatter the light their proteins emit. It cannot penetrate more than a millimeter of skin, so researchers cannot use them to look at tissues and organs deep within body.
Shapiro gets around that problem by substituting sound for light. His reporter genes create gas-filled vesicles, hollow protein cylinders filled with air. When struck by ultrasonic waves, they generate an acoustic signal. While his initial vesicles could signal gene expression in tissues, his latest techniques can resolve what is going on in individual cells.
In addition, Shapiro’s lab has developed ways to use ultrasound to trigger cells to carry out programmed functions at specific locations, like damaging cancer cells in tumors.
The work is a dazzling demonstration of groundbreaking genetic engineering, especially for someone who never planned to be a scientist.
MRIs
Shapiro arrived at Brown University in Providence, R.I., in the late 1990s. He wanted to make a difference in the world through public service and set out to study political science and philosophy. This was when the Internet was just breaking out, and Shapiro left school in his sophomore year to start an online business. Like so many other dotcoms, it went under, but not before it led Shapiro to John Donoghue, a neuroscientist at Brown.
Donoghue is a pioneer in brain electrodes, which monitor neuron behavior in laboratory animals. Ultimately, he hoped to one day to develop similar implants to enable paraplegics to control prosthetic arms and legs with thought alone.
Donoghue wanted to start a company to make the electrodes available to other researchers but knew nothing about business. He was introduced to Shapiro and asked him to help commercialize the technology.
Along the way, Shapiro had a revelation: “By pitching venture capitalists, I realized that the exciting part of story was the science—how to extract and decode signals from brain,” he said. “So, I decided to become scientist myself, and switched my major to neuroscience.”
Yet Shapiro found his efforts to probe the brain frustrating: “I remember thinking, ‘Wow, how crude is this, sticking electrodes into brains. And light can’t penetrate more than a millimeter of tissue.’
“That got me thinking about magnetic fields or sound waves, which can penetrate deep into tissue without harming cells the way X-rays do. I didn’t see enough engineers developing those technologies, so I decided that I would become an engineer and create them myself.”
That led him to MIT’s new graduate biological engineering program, where Shapiro invented a way to more directly connect MRI scans to neural activity. Whereas conventional MRI looks at changes in blood flow, he developed a contrast agent that turned on and off when the brain released neurotransmitters like dopamine and serotonin.
Yet MRI imaging posed problems. The scanners are too large and expensive for most labs, and the contrast agent he developed was not well expressed by cells in the brain.
At the time, Shapiro still thought of ultrasound as a pedestrian technology for imaging babies. Then, as a post-doc at UC Berkeley, Shapiro came across a paper describing the multigene clusters cyanobacteria used to make gas-filled vesicles. These hollow protein structures enabled bacteria to float in water so they could capture sunlight for photosynthesis.
The paper intrigued Shapiro. First, the smaller a gas-filled structure, the more unstable it tends to become. Yet nature had found a way to make nanoscale vesicles. He found it hard to believe and wanted to learn more about them.
As he did, he realized something else: the gas-filled vesicles would have very different magnetic and acoustic properties than the cell’s surrounding liquid. Perhaps he could create reporter genes that expressed vesicles that would be visible deep in the body.
The prompted him to take another look at ultrasound. “It was simpler technology,” he said. “For $2,500, I can now buy an ultrasound system that hooks into my smartphone. It has spatial resolution of 100 microns, and we can acquire images in milliseconds. Both are better than an MRI. No one was working on this. I figured that if I didn’t do it, no one else would,” Shapiro said.
But to create those reporter genes, Shapiro first had to first do some ground-breaking genetic engineering.
Complex genetics
“When we started this work in 2013, a lot of people questioned whether we could actually move vesicles genes into cells that don’t ordinarily express them,” he said.
Skeptics had good reasons to doubt. It takes only one gene to make fluorescent reporter proteins. Moving that gene from DNA located in the nucleus of a jellyfish to the DNA of another animal was fairly straightforward.
That was not the case for gas-filled vesicles. Cyanobacteria had to express nine separate genes in just the right sequence and ratios to make the proteins needed to assemble the vesicles.
More dauting, however, was that cyanobacteria and mammalian cells handled genetic information very differently. Mammalian cells, or eukaryotes, store genetic information in their nuclei. Cyanobacteria, or prokaryotes, have no nucleus. Shapiro had to not only transfer nine separate genes but find a way to make them work in a cell that used significantly different mechanisms for expression.
He started by picking a simpler problem: transferring the genes from cyanobacteria to another prokaryote, E. coli, a common gut bacterium. This involved engineering a cluster that included all the genes needed to make the vesicle proteins and the proteins that would assemble them.
After a great deal of trial and error, Shapiro’s team showed it could produce the vesicles in E. coli. Yet they were too small to respond well to an acoustic signal. To make them larger, his lab substituted genes from other species that formed larger gas-filled vesicles. The new reporter genes worked better. In 2018, his lab showed they could be used to image E. coli in the gut of a mouse.
Now. they needed to move it into mammalian eukaryotic cells.
“All bets were off because the process was so different,” Shapiro said. In bacteria, he explained, the genes all lay back-to-back on single strand of genetic material. When a cell expressed that gene, it created everything needed to build the vesicle all at once.
Mammalian cells, on the other hand, tend to express genes individually. That meant the lab needed to dial in the exact ratios of bricks and cranes needed to build the vesicles. It would require groundbreaking science. “That was something we just didn’t know if we could really do,” he said.
Fortunately, nature had solved the problem. Through evolution, viruses had mastered the art of stringing genes together to express proteins in the right ratios in eukaryote cells.
“We borrowed some viral elements that other synthetic biology researchers had been adapting to string together these bacterial genes,” Shapiro said. “Then when we put the resulting gene cassette into human cells, we got gas vesicles to form inside those cells. Of course, there was a lot of trial an error. I think the graduate student who led this project did close to 1,000 tests before he was done.”
A new type of imaging
“Since then, we’ve gotten much more sophisticated,” Shapiro said. “We’re on versions 2.0 of both our bacterial and mammalian genes. We’ve taken genes from other species that were not part of our initial set of sources and optimized their ratios and the way we express them still further.”
The team has also developed a new technique to visualize gene expression in individual cells. This involves developing vesicles that explode when hit with ultrasonic energy. Like a balloon popping, the exploding vesicles make a sound that is clear enough to resolve down to individual cells.
The result is a new type of imaging, one that reaches deep under the skin with unprecedented sensitivity, so researchers can see how cells, tissue, and organs behave in living organisms. Yet the lab needs to do more work before his technology is ready for others to use. Shapiro hopes the Hughes Medical Institute’s $9 million grant will help him meet two big items on his agenda.
The first is to make the technology so good, every biology lab in world will want to do use it. “To reach that goal, we must make this really robust and really simple to use,” Shapiro said. “Then we have to go out and teach the world how to use it. We have to show them that acoustics are as simple as optics.”
The second is to create reporter genes that sense the dynamic processes going on within cells. For example, his group recently developed a gas-filled vesicle that changes how it resonates after an enzyme makes a cut on its surface. This would signal when such enzymes are active.
They are also developing vesicles that sense calcium, an ion used by neurons to communicate with one another. “With fluorescent proteins, we could only see a few hundred microns into the cortex, the outer layer of the brain,” Shapiro said. “Or we could insert a thin optical fiber into the brain and see what happened next to it. With acoustics, we may be able to visualize an entire mouse brain all at once.”
Gas-filled vesicles might also one day play a role in medicine. His lab recently published a study showing it could deliver vesicles to tumors using genetically engineered tumor-finding bacteria.
“They would establish a little colony in the middle of the tumor,” Shapiro said. “Then we would focus ultrasound on them to blow up the vesicles and damage the tumor. The immune system would then discover this damage and attack the tumor. When we combined this approach with drugs that revved up the immune system, it led to enhanced survival for the mice in our study.”
He is also engineering temperature-sensitive cells to seek out tumors. Existing therapies often use engineered materials that seek out tumors, but as the medication moves through body, it often kills healthy as well as cancerous cells. Aiming ultrasound energy directly at the medicine-filled cells clustered around the tumor, he could direct them to release their medications only in the right location.
It is a dazzling future, and in many ways, it brings Shapiro back to his original goal of public service: “I was originally interested in politics because I wanted to make a difference in the world. But I’ve realized that science can have an even more lasting impact on humanity than politics.”