How Do Sensory Experiences Shape Brain Circuitry?
by Sally Johnson
Patrick Kanold studies cortical circuits underlying sound processing to explore their function and influence on brain development and plasticity
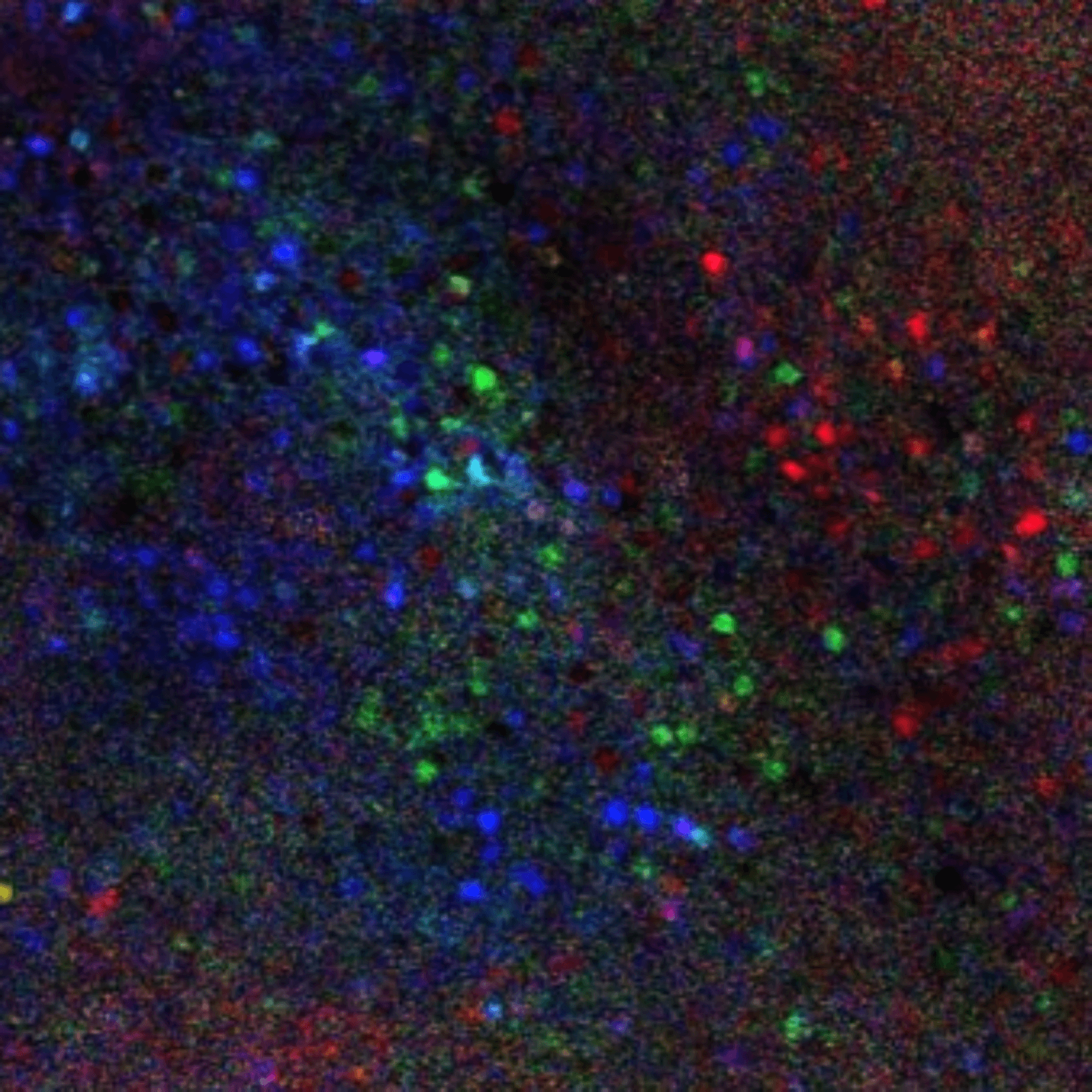
The Author
The Researcher
Understanding how brain circuitry adapts to its sensory environment can help neuroscientists explore brain function. Patrick Kanold, professor of biomedical engineering at Johns Hopkins University and a member of the Kavli Neuroscience Discovery Institute, is applying his electrical engineering education and skills designing electronic microcircuitry to study circuits underlying the functional architecture of the auditory cortex and circuits involved in plasticity of both very young (fetal and neonatal) and adult brains.

Kanold made the jump from electrical engineering to neuroscience after becoming intrigued by the intricacy of neuro circuits and their complexity. Since we don’t have enough genes within the genome to code for all of the connections within the brain, sophisticated programs must be present to enable these circuits to develop to process sensory information appropriately.
His lab is currently working with mice to try to figure out when exactly the earliest sensory experience occurs during early development—and how this experience shapes brain circuits and the subsequent development trajectory.
“This is during the fetal stage in humans, but to understand how the brain circuitry adapts to sensory experiences, we first need to figure out how we normally process information,” says Kanold. “So we’re also exploring adult brains to try to crack the neural code of how our neurons as a collective are representing and processing information to make decisions.”
In earlier work, Kanold’s lab showed that mammalian subplate neurons play a key role during cortical development and plasticity. Subplate neurons are among the first to develop within the most complex part of the brain the cerebral cortex, and make connections between a gateway within the brain for sensory information, the thalamus, and middle layers of the cortex, before eventually dying off during development.
“It was always thought these early periods of experience-dependent changes started at a certain point of development where connections between the thalamus and the middle layers of the cortex are becoming established,” Kanold says.
His lab also previously mapped the circuitry of subplate neurons in mice and ferrets, and discovered that subplate neurons can receive electrical signals related to sound before any other cortical neurons.
This year, Kanold’s lab discovered that a sensory experience—sound—can drive activity within the part of the cortex that processes sound and actually change wiring patterns much earlier than expected in newborn mice, even before their ear canals open 11 days after birth and before the thalamus activates the middle layers of the cortex.
“We’re now going much further to see if there is a specific influence on particular kinds of experiences,” he says. “For example, in humans, what happens if a baby is exposed at a particular stage to a certain kind of music as a very early experience before they were exposed later to babble or their parents’ vocalization? Many parents play music to their unborn children with the hope it will make them smarter. The question is: does it?”
It appears to be changing circuits, but it’s unknown if this is beneficial yet. “But now we’re trying to expose animals to very early stimuli to see if it changes later behavior or plasticity within these circuits,” Kanold says. “Is there a sort of preprogramming that sets the boundaries for what can be experienced later? We don’t know yet. It’s a difficult experiment because we’re dealing with very young, small animals.”
For their work with adult animals, they use all optical methods, including optogenetics—using laser light to control brain cells genetically engineered to respond to specific wavelengths—in vivo, to probe circuits and relate circuit connectivity to its function.
In adult animals, researchers can use viruses to insert genes into cells, but this often requires days or weeks. One of the main challenges is that there isn’t enough time to use this technique for a developing animal.
So they need to turn to other approaches, such as transgenic animals—with DNA from another sources inserted into their DNA. “Working with young animals is much trickier than working with adults,” Kanold points out, because “it’s a very small animal that needs to be held very stable under a microscope.”
One of the most surprising aspects Kanold’s work is revealing is just how little is known about the young brain. There’s a misconception that it’s a smaller version of the adult brain, “but it’s completely different and the rules are different,” he explains. “We know very little about the basic circuitry, changing circuitry, and the rules of how synapses change. Everything, including processing is different and slower. We almost don’t need a hypothesis because most everything we find is novel.”
On the other end of the spectrum, Kanold’s lab is working with Dr. Hey-Kyoung Lee at JHU to explore how adult sensory experience can reshape our brain. For this work, they deprive animals of visual experience and then look at the auditory system. The goal is to figure out what’s going on and whether short-term visual deprivation as an adult can change hearing.
“We found that indeed it can, and we’re working on nailing down the circuits and mechanisms that allow us to repurpose our cortex—depending on the sensory environment we find ourselves within,” Kanold notes. “This has a lot of implications potentially for a variety of sensory disorders. If you can cue up the auditory system by sensory deprivation, if you have auditory problems, speech or language problems, there may be therapeutic uses for these types of deprivations.”
The overall theme of Kanold’s work is exploring how experiences shape brain circuits, so he’s also similarly looking at aged animals to see how training paradigms can be changed for the aging brain to perhaps prevent or recover more “youthful” function. “I am aging, we’re all aging, so it’s very close to my heart,” he jokes.
The coolest implication of this work is that it may be possible to use all of these experiences to pre-shape the brain or rewire it. “If sensory experiences can shape the brain wiring of the mouse cortex, maybe this will also work in humans,” Kanold says. “Trying to develop training paradigms to perhaps reverse or prevent changes would be very powerful. It could also be useful to bring some of this into the clinic to help preemies so all of their learning processes that get disrupted by premature birth can actually happen later on.”