Experiment Probes Nature of Space and Time
(Originally published by The University of Chicago)
April 18, 2016
For the past year at Fermilab, an instrument called the Holometer has been probing the fundamental nature of space. The experiment uses an array of lasers and mirrors to try and answer the question, “If you look at the infinitesimally small scale, is space smooth and unbroken—the way we experience it in everyday life—or is it pixelated like the image on a TV screen?”
Recent initial results are homing in on an answer. Either way, the outcome will have important consequences for physical theory. It may even help address one of the most nagging problems in physics: how to reconcile Einstein’s General Theory of Relativity with quantum mechanics.
The Holometer is an anomaly—an experiment in a scientific territory in which there are no other experiments. Craig Hogan, professor of astronomy and physics at UChicago and head of Fermilab’s Center for Particle Astrophysics as well as a member of the Kavli Institute for Cosmological Physics (KICP), envisioned the Holometer as a way to probe the zone where quantum mechanics and general relativity might come together.
General relativity describes space-time and gravitation in the large-scale world we inhabit. It makes definite predictions. Quantum mechanics describes the world at the atomic and sub-atomic scale, which is not continuous but granular, or quantized. It deals in probabilities, uncertainties, and there is a limit to the amount of information that can be had about anything being observed. It has other stranger qualities as well, such as the fact that distant parts of a quantum system can be influenced by each other—a phenomenon that Einstein pejoratively dismissed as “spooky action at a distance.”
Each theory works beautifully in its own realm, but scientists have not discovered a theory of quantum gravity that unites the two. And yet, at an incredibly tiny scale called the Planck length—about 10-35 meters—“We know that gravity runs into quantum mechanics, no matter what you do,” says Hogan.
The problem is getting from one scale to the other. In the canonical way of doing science, theory makes predictions, experiments test those predictions, and experimental results inform the next iteration of theory. But in the domain of quantum gravity, there is no experimental evidence at all. In fact, there is no real theory—only competing models.
“Many of these ideas are great ideas,” says Hogan. “It’s just that people don’t know which ones apply to reality. And there’s no experiment to guide them.”
That’s where the Holometer comes in. Its mission is to look for “holographic noise”—an effect of quantum uncertainty in our 3D universe. Hogan’s idea is that if space itself were quantized at the Planck scale, our apparently continuous space could emerge from it in much the same way that fluid, apparently continuous air emerges from the bouncing around of discrete molecules. But some of the strange quantum behavior should “leak out” from the Planck domain into our large-scale world. And that leakage is what the Holometer is designed to detect. It is exploring and measuring space at the Planck scale—something that has never been done before.
The search for quantum jitter
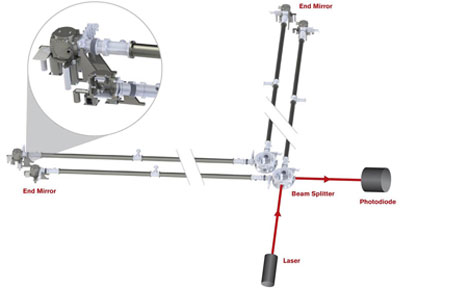
Measurable holographic noise could take a couple of different forms—quantum jitter or purely rotational quantum twists of space. Recently, the Holometer definitively eliminated the first possibility: There is no quantum jitter in space. News media around the world reported that the finding means there is “no evidence that the universe is a hologram,” but Hogan says the work’s broader significance is the window it opens into phenomena at the Planck scale.
“That’s an important result,” Hogan says. “It sets a limit on something nature could have been doing. The other part of the news is that we have this technique now that works.”
The technique involves a version of a tool used in physics since 1887: a Michelson interferometer, named for the former UChicago physicist and Nobel laureate Albert A. Michelson. Actually, the experiment isn’t one interferometer but two identical instruments set close together. Each comprises two perpendicular 40-meter arms, each with a mirror at the end, that intersect at a beam-splitting mirror. Laser light sent through the beam splitter splits, half going down one arm and half down the other. Both beams are reflected back through the beam splitter, where they recombine, or interfere.
If there had been a quantum jitter in space at the Planck scale, the relative positions of the mirrors would have shifted ever so slightly, and the jitter would have shown up as a tiny offset in the interference pattern.
Using two instruments that share the same slice of space-time makes it possible for the scientists to exploit the quantum mechanical phenomenon of correlation to help them tease out any sign of jitter. ”If you have two interferometers next to each other, the space in one is correlated with the other,” says Hogan. “So if one moves, the other moves with it even if there’s no physical connection.” The two instruments are shielded from ambient sources of jiggle and isolated from one another so that no light can move between them.
“They really had to be independent systems,” says Stephan Meyer, professor in astronomy and astrophysics and physics at UChicago and a KICP member who designed most of the Holometer’s electronic and mechanical systems. “If you have two devices and all of the jiggling that they would normally do is not related, you can use a mathematical technique that sniffs out the part where they move together.”
Unique experiment guiding theory
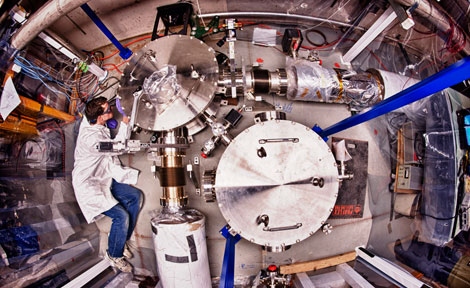
The amount of movement is unimaginably tiny—almost a billion times smaller than an atom. Through an iterative process of multiplication and averaging, the minute shared signal from the Planck domain rises up through the data as the mundane noise from other sources averages away.
That the Holometer exists at all is remarkable. The physical space and technical systems required to build and house it don’t exist on a university campus. “I don’t think we could have done it anywhere but Fermilab,” says Hogan. Equally important, the theoretical question it explores, while potentially important, is “off to the side” of the current mainstream, he says. And it is risky. “You have to be in an unusual environment to enable that,” he says. Meyer agrees. “It requires a special place,” he says. “Chicago has departments that are extremely broad-minded, and it’s that open-mindedness that makes it possible to try these things.”
The Holometer has ruled out one source of holographic noise. But even though space doesn’t jitter, it remains possible that it twists. So the next step is to reconfigure the apparatus into a different shape so that it can look for rotational movement. “I really want to know the answer to whether the universe has quantum twists or not,” says Hogan.
But he’s encouraged by the project’s findings so far. “In a way, for me, this thing has already succeeded exactly as we hoped, because it’s guiding theory. We didn’t find jitter, so it’s the other possibility, if at all. So it worked; it’s constraining the range of possibilities.”