Dwarf Galaxies Loom Large in the Quest for Dark Matter
by Adam Hadhazy
A batch of newly discovered satellite dwarf galaxies orbiting the Milky Way should help scientists better grasp the evolution of the universe while also honing in on dark matter's identity.
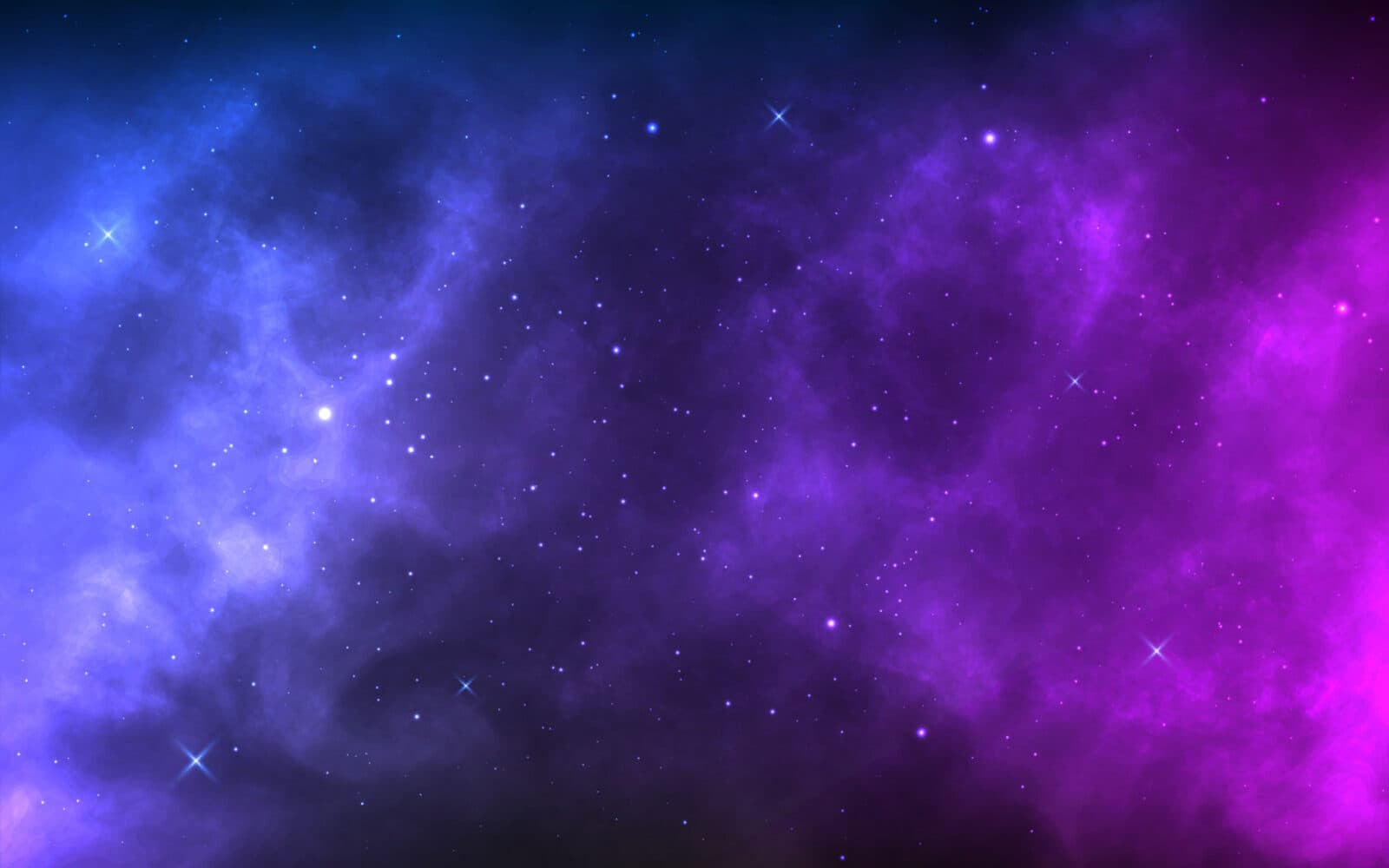
The Author
The Researchers
IN ITS INAUGURAL YEAR OF OBSERVATIONS, the Dark Energy Survey has already turned up at least eight objects that look to be new satellite dwarf galaxies of the Milky Way. These miniature galaxies – the first discovered in a decade – shine with a mere billionth of our galaxy's brightness and each contains a million times less mass. Astronomers believe the vast majority of material in dwarf galaxies is dark matter, a mysterious substance composing about 80 percent of all matter in the universe. Dwarf galaxies have therefore emerged as prime targets for gathering potential clues about dark matter's composition.

Some theories suggest dark matter particles and antiparticles should produce telltale gamma rays when they collide with each other. Accordingly, scientists used the Fermi Gamma-Ray Space Telescope to study the newfound dwarf galaxy candidates, as well as a group of dwarf galaxies already on the books.
The telescope detected no significant gamma-ray signals from either set of dwarf galaxies, however, leaving scientists still in the hunt for dark matter. Four studies earlier this spring from the Dark Energy Survey and Fermi announced this flurry of results.
On May 18, 2015, The Kavli Foundation spoke with three astrophysicists about the continuing search for dark matter data in space and how dwarf galaxies can help us understand the evolution of our universe.
The participants were:
- JOSH FRIEMAN, the Director of the Dark Energy Survey at the Department of Energy's Fermilab Center for Particle Astrophysics, a Professor of Astronomy and Astrophysics at the University of Chicago, and a member of the Kavli Institute for Cosmological Physics (KICP).
- ALEX DRLICA-WAGNER, a Schramm Experimental Fellow at the Fermilab Center for Particle Astrophysics and a KICP Associate fellow.
- ANDREA ALBERT, a Research Associate at the SLAC National Accelerator Laboratory and the Kavli Institute for Particle Astrophysics and Cosmology (KIPAC) at Stanford University.
The following is an edited transcript of their roundtable discussion. The participants have been provided the opportunity to amend or edit their remarks.
THE KAVLI FOUNDATION: What were your first thoughts when you saw the signs of these dwarf galaxies in Dark Energy Survey data?
ALEX DRLICA-WAGNER: It was very exciting and definitely not something you get to experience every day. It was obvious from the start that some of the objects in the data were real stellar systems and very probable that they were new dwarf galaxies.
JOSH FRIEMAN: We released the first year of Dark Energy Survey data internally to our international collaboration of scientists in late December. Within a week or two, Alex and his co-lead author, Keith Bechtol, who is a postdoctoral research fellow at the Kavli Institute for Cosmological Physics, helped to lead the group of Dark Energy Survey scientists who identified these objects. Alex and Keith had spent a long time developing methods for detecting dwarf galaxies in this data, so when it became available they were instantly able to jump on it.
DRLICA-WAGNER: When you see something like this emerge from your data analysis for the first time, that's very special. You've invested so much time going through all of the data, you can feel a little lost down there in all the details.
ANDREA ALBERT: Yeah, I know how that feels! [Laughter]
TKF: How did the Dark Energy Survey enable the discovery of these dwarf galaxies?

FRIEMAN: We didn’t actually build the Dark Energy Survey to do this. These nearby dwarf galaxies are foreground objects to what we're really trying to study, which are hundreds of millions of distant galaxies. We are measuring those distant galaxies' properties and their distribution to figure out why the expansion of the universe is speeding up due to dark energy. We realized that those same data would be very useful not only for studying the distant universe, but also for identifying very faint dwarf satellite galaxies of our own Milky Way.
DRLICA-WAGNER: With the Dark Energy Camera, the principal instrument for the Dark Energy Survey, this is the first time we’ve had a super-sensitive, large-field digital camera in the southern hemisphere. The explosion of dwarf discoveries about a decade ago in the northern hemisphere was made possible because of the state-of-the-art technology involved in the Sloan Digital Sky Survey [SDSS]. With the Dark Energy Camera, we have an even better camera than SDSS and we have a whole new region of sky.
FRIEMAN: This is one of those examples where you build something with one goal in mind, but if it's properly designed it turns out to be quite useful for a variety of other scientific studies as well.
DRLICA-WAGNER: As Josh just said, the Dark Energy Survey was not intended for this kind of science, but it really presented an amazing opportunity. We were able to find this abundance of new stellar systems by combining two models of what we expect dwarf galaxies to look like. In general, dwarf galaxies have more stars near their centers and fewer stars near their peripheries. Additionally, the stars in dwarf galaxies tend to follow a very distinct pattern of color and brightness. By combining these two models, we had a much better chance of finding dwarf galaxies.

TKF: Why are dwarf galaxies so notoriously difficult to spot?
DRLICA-WAGNER: Dwarf galaxies are hard to find because they contain very few bright stars. Also, dwarf galaxies are mostly made of dark matter. If we figured out a way to see dark matter, then dwarf galaxies would be easy to see.
ALBERT: We're awesome at seeing stars because we are awesome at seeing light. Unfortunately, dark matter does not directly make light. So we call it "dark." Physicists are not that creative when we come up with names!
FRIEMAN: One thing astronomers like to do when they're presenting at conferences is to show an image of what a dwarf galaxy looks like. The presenter will show an image and it looks like nothing is there, like a random field of stars. And then on the next slide, the astronomer will circle all the objects that are members of that dwarf galaxy, and then it pops out at you.
What you really need to find these dwarf galaxies are very sensitive observations that allow you to detect faint stars and determine their colors. All that is critical for being able to distinguish a grouping of stars that together form a dwarf galaxy from the light of background galaxies and from foreground stars in the Milky Way.
ALBERT: I'd also mention that many of the reasons why dwarf galaxies are very, very challenging to detect are also the reasons why they are one of the best targets we have today for dark matter searches.
TKF: That comment segues into why dwarf satellite galaxies of the Milky Way are so scientifically valuable. Alex, you've said before that their dark matter content makes them important for both astronomy and physics. Can you elaborate?
DRLICA-WAGNER: This long-standing problem of the identity of dark matter is a topic where you historically have seen a lot of interplay between astronomy and physics. Dark matter was first a purely astronomical problem. It was originally discovered by observations showing that the force of gravity is not strong enough to prevent galaxies and clusters of galaxies from flying apart. So astronomy at the very start was sort of telling physics, "Hey, there's something here that we don't understand." In parallel physicists, were coming up with their own ideas for new fundamental particles. Eventually, people started to assemble the idea that missing "dark" matter particles could be holding galaxies together.

FRIEMAN: This notion of dark matter particles goes back 35 years or so and it's very compelling. Assume you have an unseen particle that weighs, say, 10 to 100 times the mass of a proton. Say it only rarely interacts with particles of normal matter through a force similar to the "weak force," one of the four known forces of nature. Particles with these properties would very naturally be around in the right amounts in the universe to be the dark matter that we infer in galaxies and clusters of galaxies. The particles would also possess the right properties to explain the formation of galaxies and larger structures.
DRLICA-WAGNER: This melding of ideas from astronomy and physics has really come together to make a cosmic frontier of physics. We’re trying to push our fundamental understanding of physics through a better understanding of the cosmos with observations like those from the Dark Energy Survey.
TKF: Dwarf galaxies and the search for dark matter particles are a prime example of the intersection of astronomy and physics. Andrea, your specialty is looking for the signatures of dark matter particles that hypothetically self-annihilate, rather like how normal matter particles and antimatter particles annihilate each other. This annihilation should generate telltale gamma rays. Yet the Fermi Gamma-Ray Space Telescope hasn’t glimpsed gamma-ray signatures from dwarf galaxies, including the newly discovered dwarfs, that are suggestive of dark matter. What is your reaction to this?
ALBERT: It is disappointing. I think anyone who says that it's exciting that we haven't discovered particles of dark matter is a liar. [Laughter] So yes, we have not seen dark matter yet. However, we are just starting to scratch the surface. We have calculated a rate at which we expect annihilations to occur, according to various models, and determined what the gamma-ray signature of these annihilations should be. This annihilation rate and its gamma-ray signals are tied to the mass of the dark matter particles. At this point, we have just started to test for the most promising dark matter candidates across a small range of masses. There are models for more massive dark matter particles than we can now detect with Fermi, and which are just as viable. So I think that if dark matter is hiding, we are narrowing in on it, and that's exciting.
FRIEMAN: There's a real synergy between the Dark Energy Survey, which is an optical survey of the sky, and the Fermi experiment, which is searching over the whole sky for gamma rays. It has been very exciting putting these two projects together to make progress in constraining dark matter. I agree with Andrea that even though the latest studies report a non-detection of signature gamma rays, it's nevertheless important progress in constraining what the dark matter could be. And I think it's pushing us closer to either detecting dark matter particles or having to go in a different direction to explain dark matter.
ALBERT: The search for dark matter reminds me of the history of the search for the Higgs boson, which we finally discovered in 2012 at the Large Hadron Collider [LHC] at CERN. A couple of years before then, we were looking for the Higgs boson in the less powerful Tevatron particle accelerator at Fermilab. An experiment there was one of the first to rule out certain masses of the Higgs boson. That told us we needed to go and build a better accelerator before we would actually find the thing. Fermi's been running for over six years now, but there is a new gamma-ray experiment in the making called the Cherenkov Telescope Array. It is going to be able to test for much heavier dark matter particle masses inaccessible to Fermi.
"Many of the reasons why dwarf galaxies are very, very challenging to detect are also the reasons why they are one of the best targets we have today for dark matter searches." —Andrea Albert
TKF: How else can dwarf galaxies help us pin down the properties of dark matter?
DRLICA-WAGNER: It's worth noting that there are multiple targets in the sky where you'd expect to see a gamma-ray signal from dark matter. One of the most tantalizing is the center of the Milky Way galaxy itself. Unfortunately, the center of our Galaxy is a very active region, and there are loads of gamma rays coming from there. The issue is that when you see these gamma rays, it could just be that you don't fully understand how our Galaxy's center is producing them astrophysically. Or, on the other hand, there could be a dark matter component to the gamma-ray emissions. Dwarf galaxies are extremely important because you can use them as a cross-check on any interesting signals you see from the Galactic center.
ALBERT: Interpreting this excess of gamma rays from the Milky Way's center as possibly coming from dark matter annihilations highlights how important it is to have a large sample of these dwarf galaxies. The Galactic center is close to us and it's really bright in gamma rays, whereas these dwarf galaxies are like little, dim candles. So how are we ever going to compare dwarf galaxies to the big, bright Galactic center? If we have enough dwarf galaxies, we can stack up them up statistically for a comparison, so we can then start to test and rule out models that don't fit the data.
DRLICA-WAGNER: That's right. While we will likely have a smaller gamma-ray signal from dwarf galaxies, the signal is much cleaner and easier to study than from the very active center of the Milky Way galaxy.
“We’re trying to push our fundamental understanding of physics through a better understanding of the cosmos with observations like those from the Dark Energy Survey." —Alex Drlica-Wagner
TKF: By finding these new satellite dwarf galaxies of the Milky Way, do you think we’re closer to solving the so-called missing satellite problem? According to the leading theory of Big Bang cosmology, known as the cold dark matter model, there should be hundreds of satellite dwarf galaxies orbiting the Milky Way. Yet we've only found a few dozen. Are we now starting to plug this gap?
FRIEMAN: This has been an interesting problem and it gets into the cosmological issue of the formation of structure in the universe, of how galaxies and galaxy clusters came to be. One of the exciting things about dwarf galaxies is that they are likely some of the first large structures that formed in the universe. In the cold dark matter model, structure forms hierarchically. That means that smaller structures formed first and then merged together to form larger structures, made of both dark matter and galaxies.
DRLICA-WAGNER: The population of dwarf galaxies tells us something about how galaxies form. They address larger astrophysical questions about how our galaxy came to be and how other large galaxies form from gathering up the small, original galaxies in the universe.
FRIEMAN: There is this issue, though, when you run a computer simulation of how structure forms in the cold dark matter model. A galaxy with a similar size as the Milky Way ends up with a halo of dark matter surrounding it. But this halo should have lots of clumps within it that have masses comparable to those of dwarf galaxies. The assumption is that since dark matter and normal matter clump together due to gravity, you would naively expect those dark matter clumps to be where dwarf galaxies form. In other words, the dark matter halo around our galaxy should be teeming with hundreds of dwarf galaxies or more, but we see only a few. The missing satellite problem is that there's a mismatch between the number of dwarf galaxies found and the number predicted from theory.
As we've drilled down deeper, further and fainter with the SDSS and now with the Dark Energy Survey, we are finding more dwarf galaxies. But that's only one piece of the puzzle. You also have to understand the detailed physics of galaxy formation to say whether those clumps of dark and normal matter in the Milky Way halo would actually have formed stars that we can see. There are some scenarios in which you don’t expect all of those clumps to have lit up and formed dwarf galaxies. So I would say this finding of the eight new dwarf galaxies helps inform the missing satellite problem, but it also wasn’t the problem that was keeping theorists up at night the last few years.
ALBERT: An astrophysical problem that I'm hearing people talk more about these days is the "too big to fail" problem. The problem is, why don’t we see larger dwarf galaxies? Why isn’t matter clumping on a larger scale than the dwarf galaxies, but on a smaller scale than the Milky Way galaxy?
FRIEMAN: To date, we've had roughly 25 dwarf galaxies to study with regard to the sizes they attain in the dark matter around the Milky Way. In the next few years, as we get more information on the new dwarf galaxies we've found, plus other new ones, that number will increase. That should help us quantify the "too big to fail" problem and decide how much of a problem it is.
TKF: Do you think the Dark Energy Survey will turn up more dwarf galaxies?
FRIEMAN: We found these dwarf galaxies in the Dark Energy Survey's first six-month season of observations. We'll have five of these observing seasons over five years. During the first season, we observed about 40 percent of the area of the sky we will eventually cover. In the second season, which ended in February, we observed most of the remainder of the sky area, so we are hoping for a good harvest of new dwarfs. In the subsequent seasons, we won’t cover new areas, but we will take deeper observations of the previously covered area. In principle, that can enable us to find dwarf galaxies we haven't spotted yet that have fainter or fewer stars in them.
DRLICA-WAGNER: Over these later seasons, we will also gain a better understanding of the dwarf galaxies we have already found. As for the number of new dwarfs we'll find, we'd expect to find maybe 10 more based on the area of the survey we have covered and the additional area we are adding. But there are important caveats, such as whether dwarf galaxies are distributed evenly across the sky. It's an open scientific question that we hope to address with future years of the Dark Energy Survey.
“These new dwarf galaxies are perhaps some of the earliest structures that formed in the universe and are the building blocks of the large-scale structure we see today.” —Josh Frieman
TKF: Josh, you've said that cosmology is like archeology on a grand scale. Just as an archaeologist might look at ruins or pottery shards to piece together an ancient society, we can see how galaxies are distributed in space to learn what the universe looked like in the past. Can these new dwarf galaxies help us trace back the history of the Milky Way and the universe?
FRIEMAN: You can think of these dwarf galaxies as pottery shards left over from an earlier period of cosmic evolution. These new dwarf galaxies are perhaps some of the earliest structures that formed in the universe and are the building blocks of the large-scale structure we see today. They're much smaller than the mass of the Milky Way, and some of these dwarfs appear to have stars in them that are quite old. So these dwarf galaxies are potentially an indicator of what happened in the early phases of structure formation.
The first two major results from the Dark Energy Survey are kind of poetic when viewed in juxtaposition. One major result is these dwarf galaxies. The other is a large-scale dark matter map we released in April, which shows superclusters of galaxies and dark matter—some of the largest structures in the universe that have ever been seen. So surveys like the Dark Energy Survey are giving us information over this very broad range of scales, from dwarf galaxies to superclusters, that add to our picture about how structure has formed and evolved over time. And that’s important also for the main thing that we want to do with the Dark Energy Survey, which is understand why the expansion of the universe is speeding up due to dark energy. There's a grand competition taking place on the largest scales of the universe between gravity trying to pull things together and dark energy trying to push things apart. Understanding how structure formed and evolved over time is one of the tools that we will use to figure out the nature of dark energy.
TKF: Alex and Andrea, do you guys also feel like cosmic archeologists? Or maybe more like cosmic bounty hunters trying to capture dwarf galaxies and their signals of dark matter annihilation?
ALBERT: I feel like a cosmic Sherlock Holmes. All of the dark matter analyses I've been involved in have not seen anything, so it feels like dark matter is just out of my grasp. Dark matter is kind of like my Moriarty.
DRLICA-WAGNER: My thesis was similar to Andrea's work searching for dark matter annihilation in gamma rays. One thing that I've learned from the recent discoveries in DES is that it is really, really fun to find things. I can only imagine how extraordinarily exciting it will be if and when we find definitive evidence of dark matter.
ALBERT: It would be a bigger finding than the Higgs boson. It would be evidence for physics beyond the Standard Model of particle physics that is a great description of the 20 percent of normal matter in the universe which actually only makes up five percent of the grand total of the total mass and energy in the universe.
FRIEMAN: Yes, we can say we have a really, really good understanding of five percent of the universe. [Laughter]
ALBERT: There’s a quote that resonates with me and my feelings toward finding dark matter that people attribute to Confucius: "The hardest thing of all to find is a black cat in a dark room, especially if there is no cat." Fortunately, at this point, I don’t think we’ve searched the entire room!