Where Do the Electrons Go?
by Zhuoying Lin
Identifying the current path in a quantum anomalous hall insulator
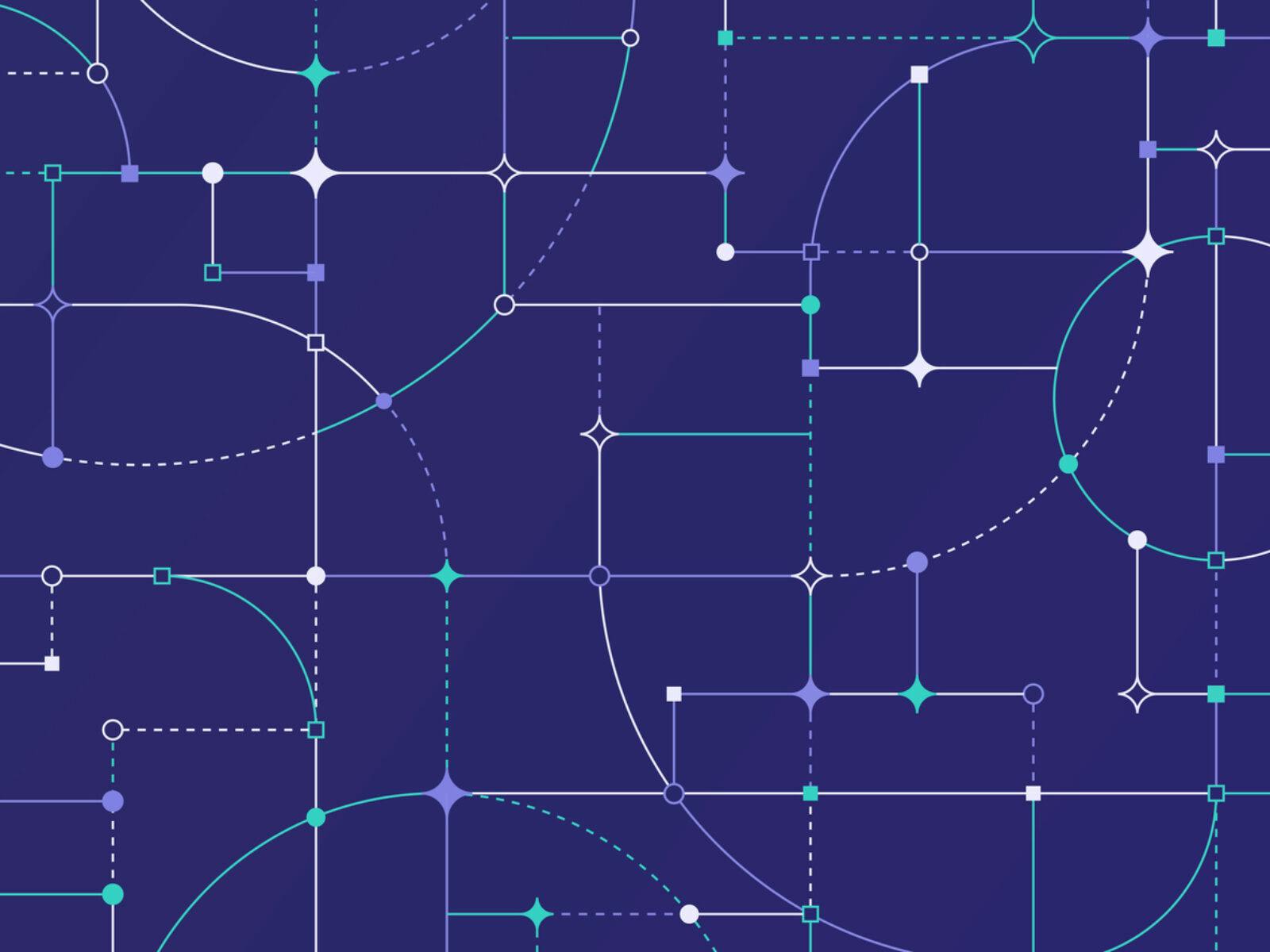
The Author
Electrons can flow in insulators? This is not new in quantum anomalous hall (QAH) insulators, but when it is found to flow in the interior rather than the edge of the materials, this is surprising.
Using highly sensitive magnetic imaging, Professor Katja Nowack at Cornell University and her team reported the unexpected electron behavior in the commonly studied topological material chromium-doped bismuth antimony telluride, in which the quantum anomalous hall effect was first observed in 2013. Their research “Direct Visualization of Electronic Transport in a Quantum Anomalous Hall Insulator” was published in Nature Materials.
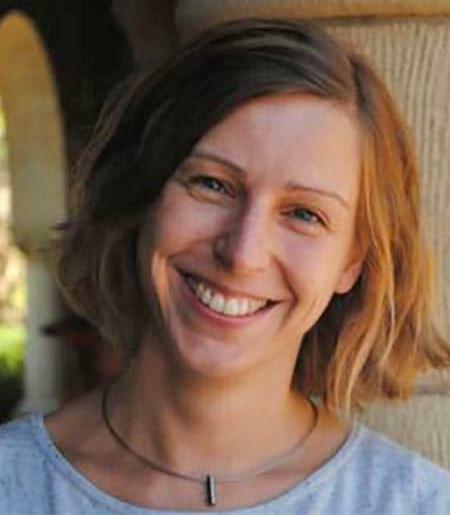
Topological insulators are special in being conductive along the edge but insulating on the inside even without an applied magnetic field. Such phenomenon is similar to the Quantum Hall effect, in which the material resistance is quantized to discrete values, but only in a large applied magnetic field. Meanwhile, topological insulators can achieve the same effect in zero magnetic field if one can magnetize them such as chromium-doped bismuth antimony telluride in which the chromium dopants provide the magnetization. These are called quantum anomalous hall insulators.
Electrons in quantum anomalous hall insulators travel in a single direction without interference to each other, resulting in current with minimal energy loss that is dissipationless, similar to superconductors. This unique electron property makes quantum anomalous hall insulators exceptionally promising for next-generation quantum devices.
“Topological materials are quite fascinating to study, and the quantum hall effect is one of the most mind-blowing solid state effects I know,” Nowack said. “Yet there’s a long-standing debate on where the electrons flow microscopically.”
To visualize the current distribution in their material, the research team used the scanning superconducting quantum interference device (SQUID) sensor, an extremely sensitive tool that can detect small magnetic field at temperatures as low as 25mK (-273°C) and is available only at a handful of labs around the world. The SQUID sensor is mounted on positioners that raster it across a sample such that the SQUID acquires an image of the local magnetic field produced by the current. From that image of the magnetic field the researchers obtain a map of the current density.
In contrast to the common belief that current will only flow through the edge, the result shows that there is current passing through the bulk of the topological material.
“We give a proof of existence that the current can flow through the bulk and challenge the simple picture of edge conduction.” Nowack said. “There is no universal behavior of the current flow, it is more complicated than that.”
The team found the current behavior depends on the applied voltage and, interestingly, there are also variations in current distribution from sample to sample. This suggests that the current path can also be affected by the fabrication process that ultimately changes the local electrostatics of the material.
“It will be interesting to perform more statistical studies on different samples as well as different topological materials to better understand the interplay between magnetic landscape and current distribution,” Nowack commented on the future steps. “There is also a material challenge to push the working limit of topological materials to higher current and temperature for application purposes, including engineering and discovering new materials with quantum anomalous hall effect. I believe that our kind of imaging can have a real impact there.”